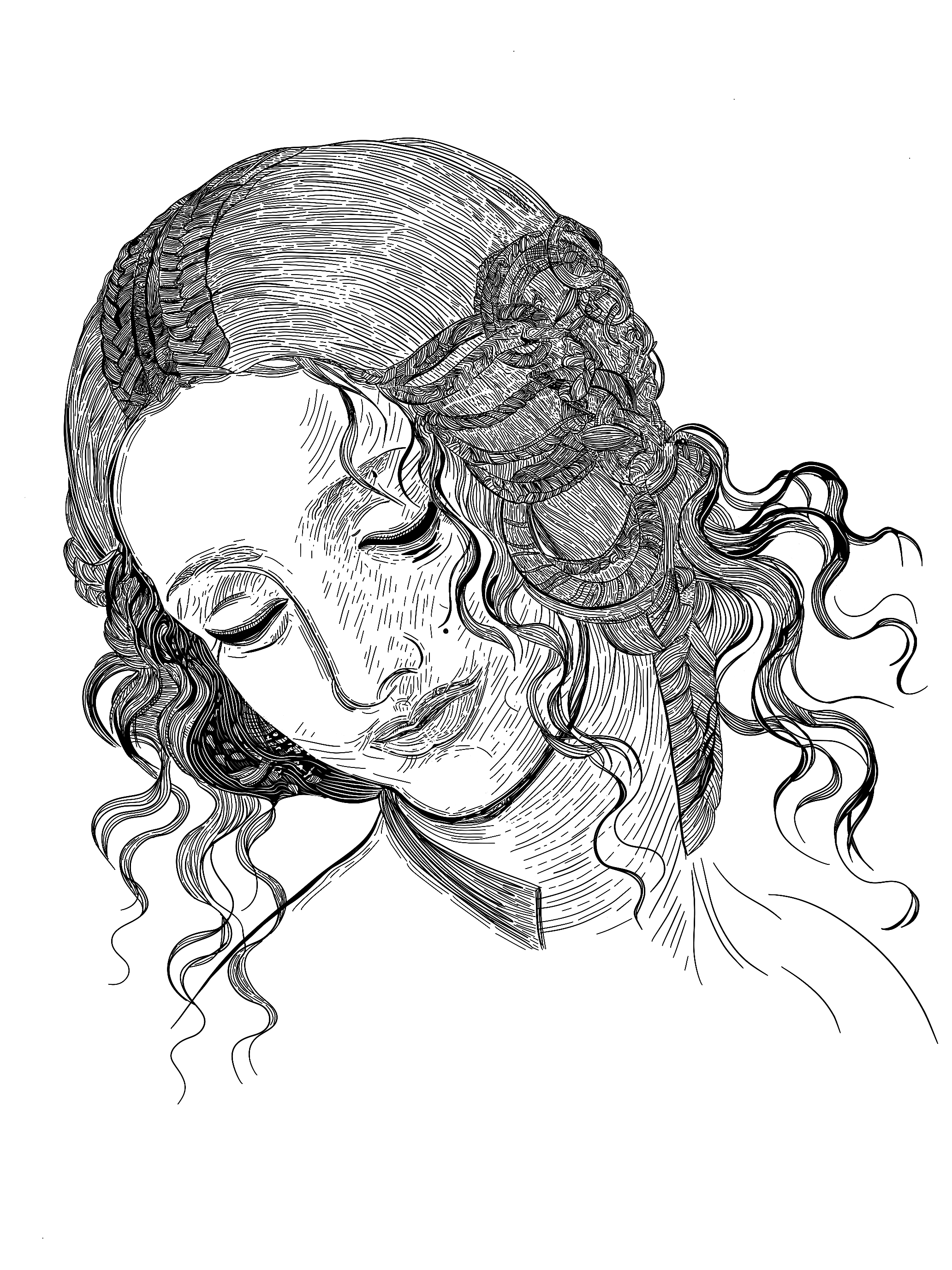
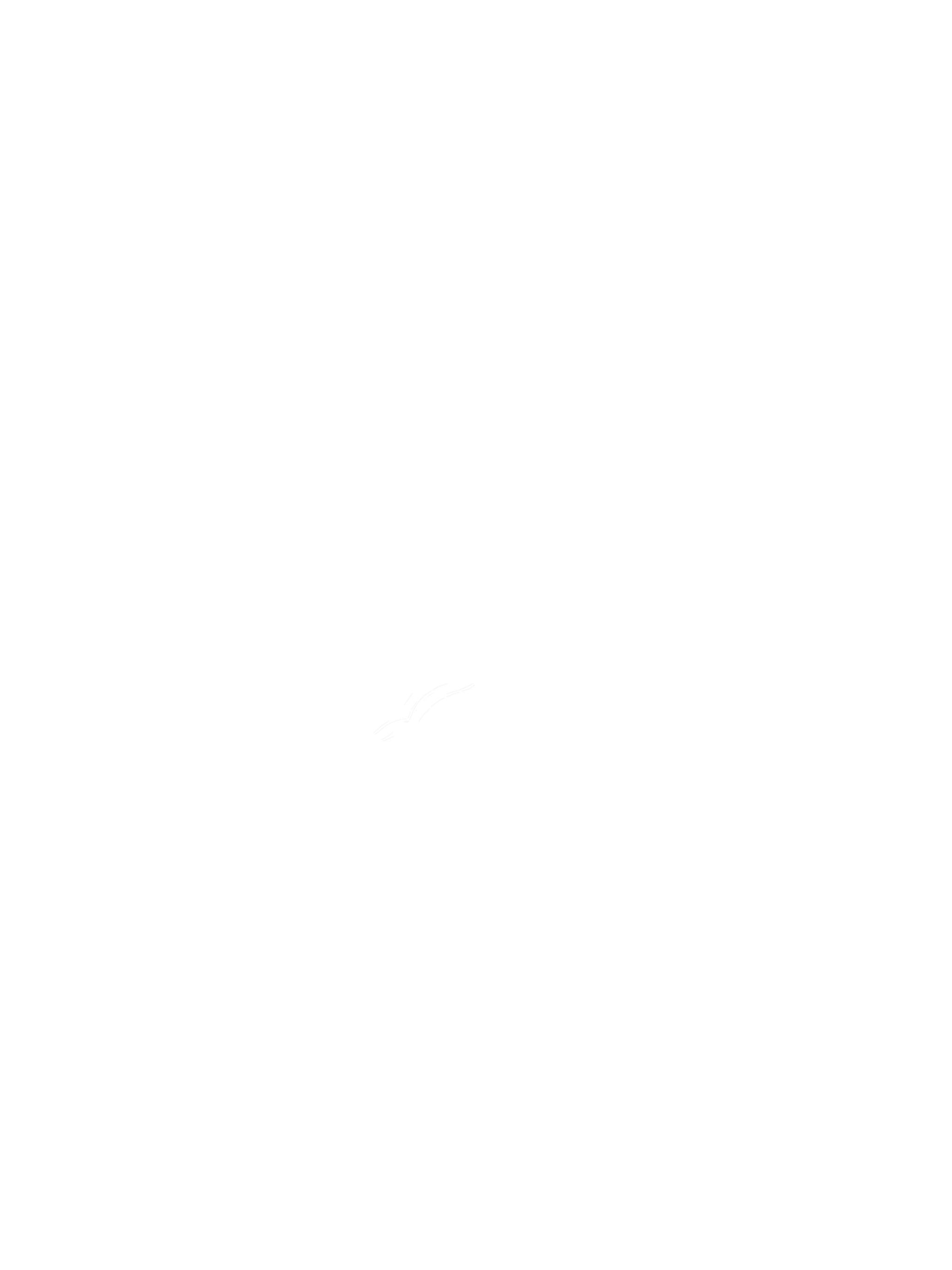
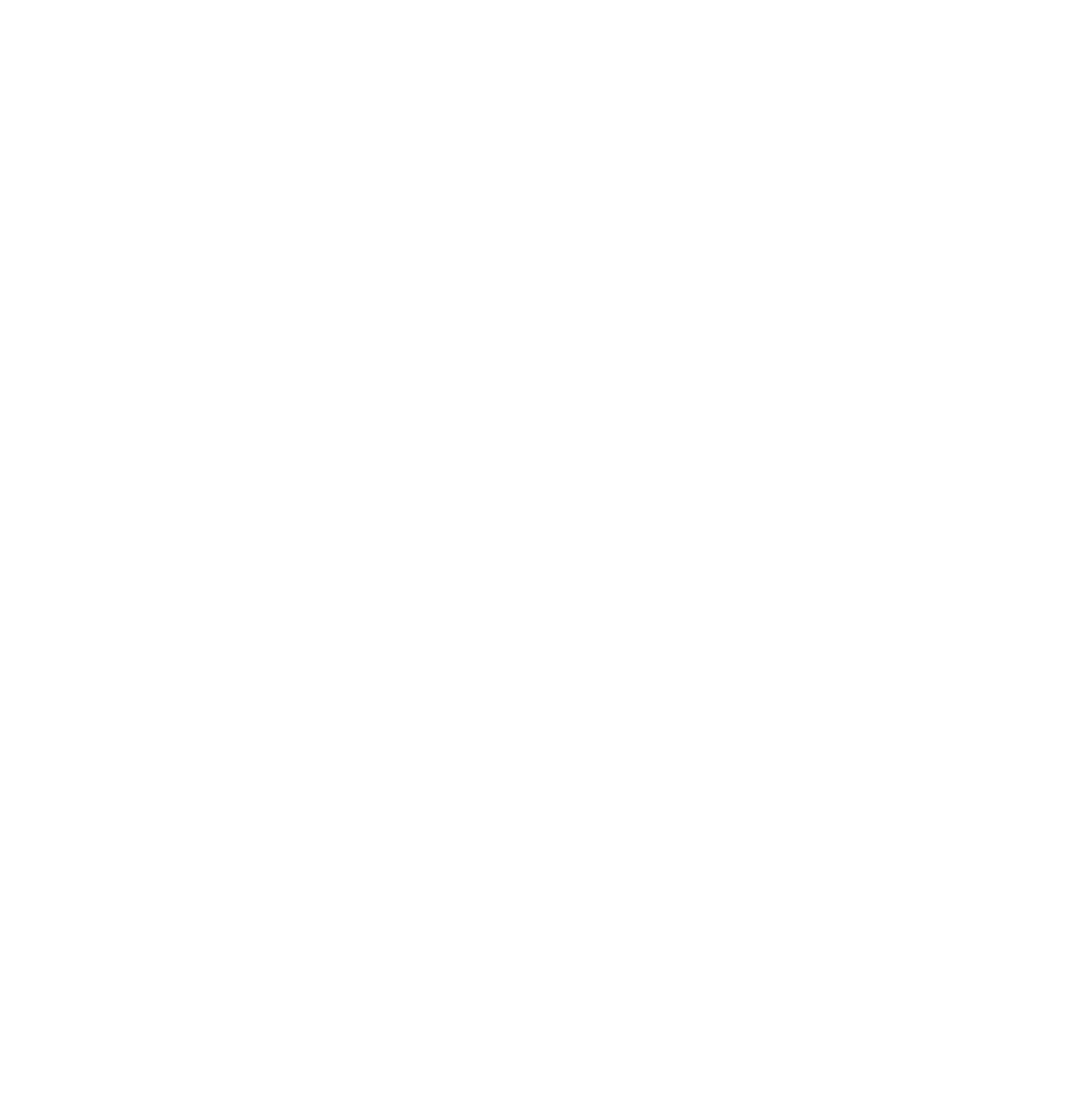
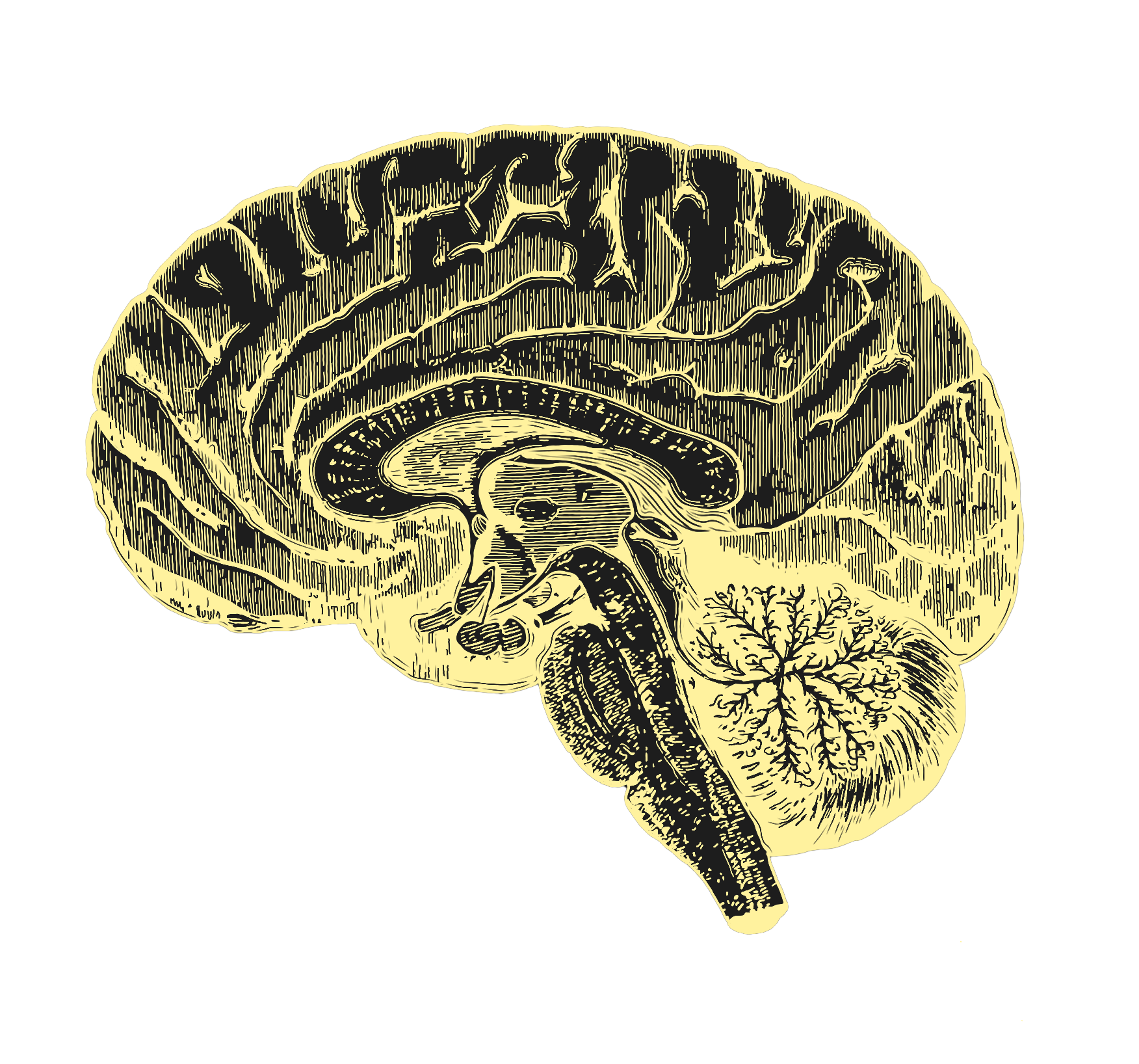
fails with age.
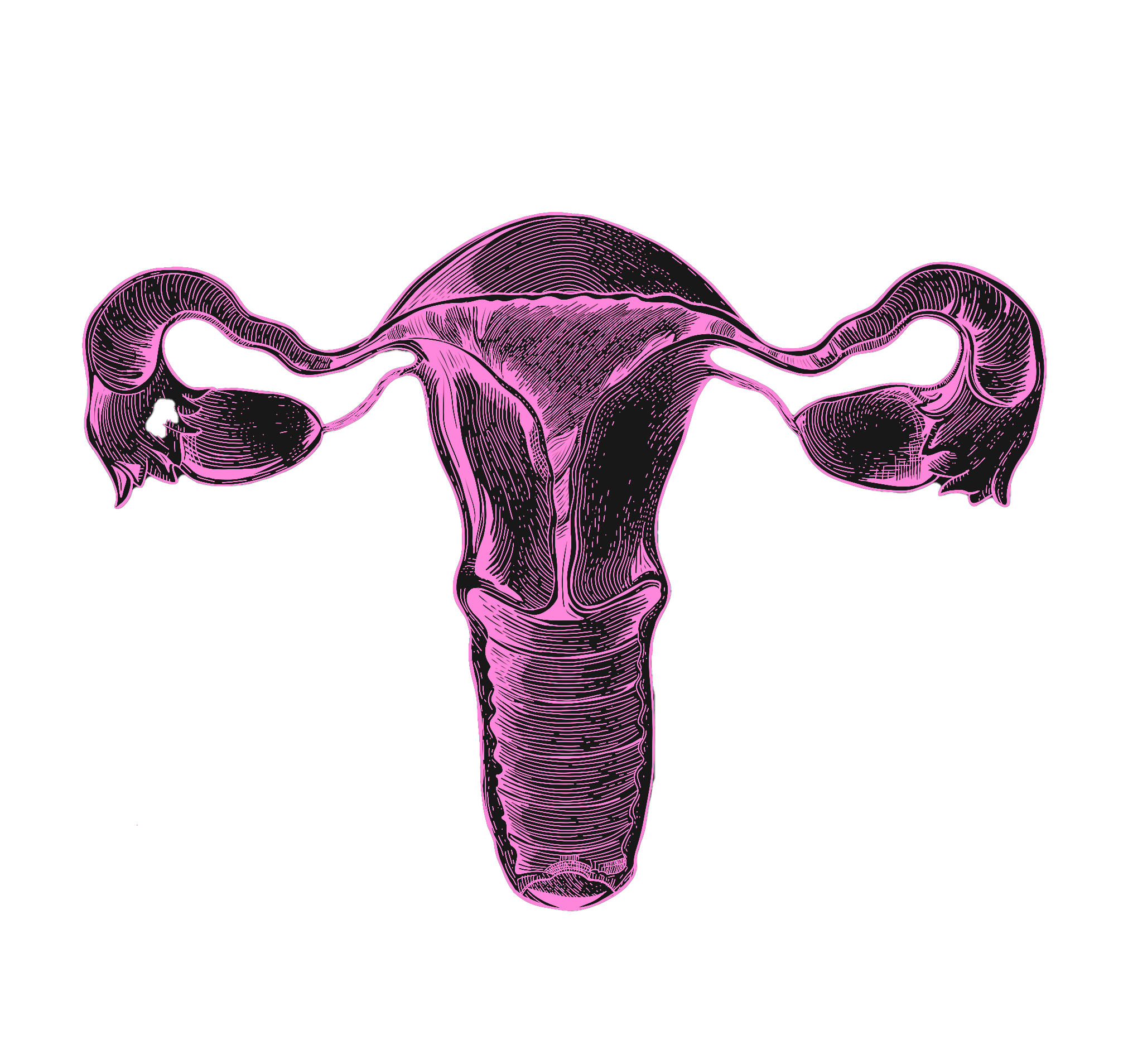
disappears by age ~40.
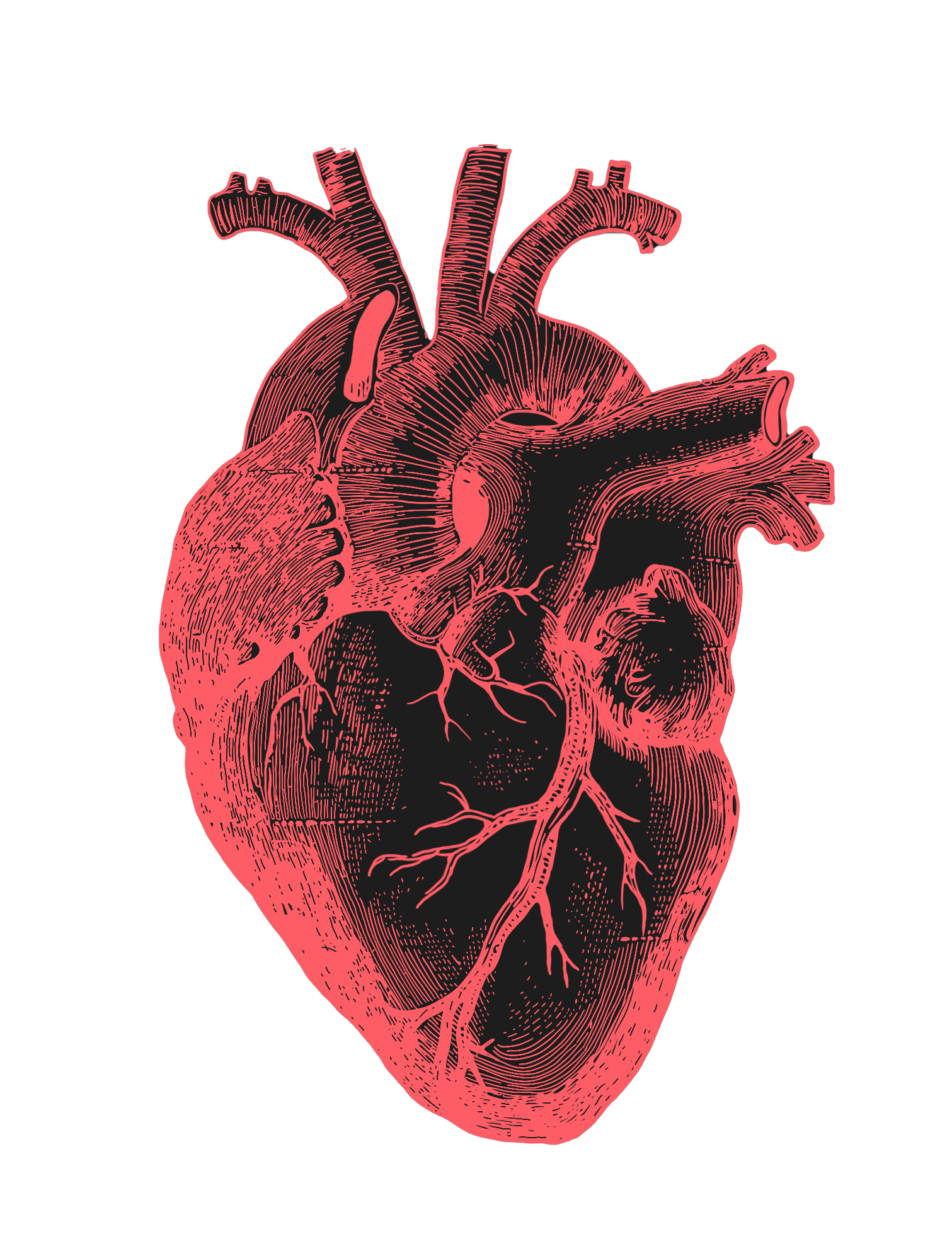
their organs replaced as they age.
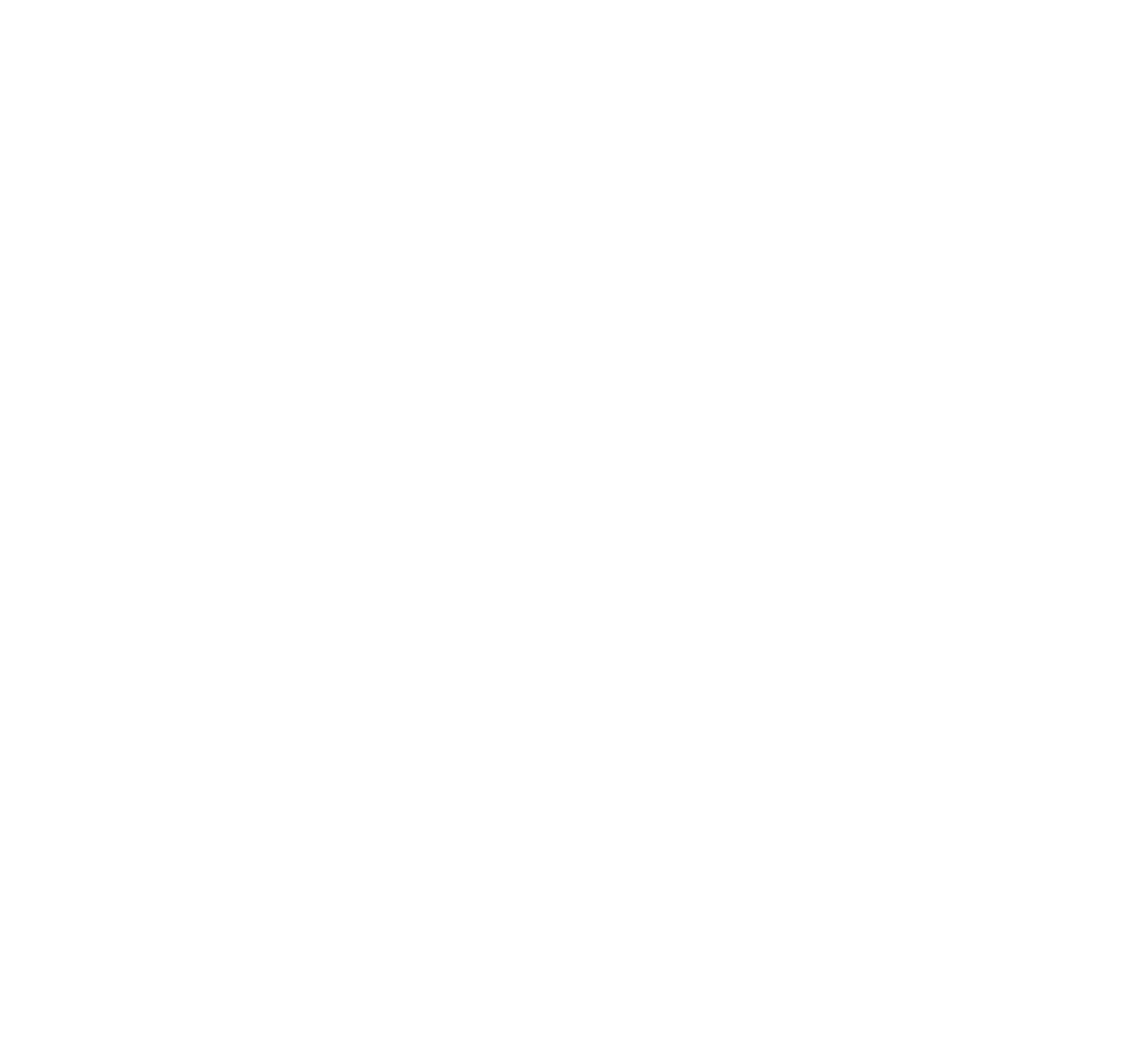
aging by 1 year
to U.S. GDP
return Net Present
Value over decades
or gained
by 2050
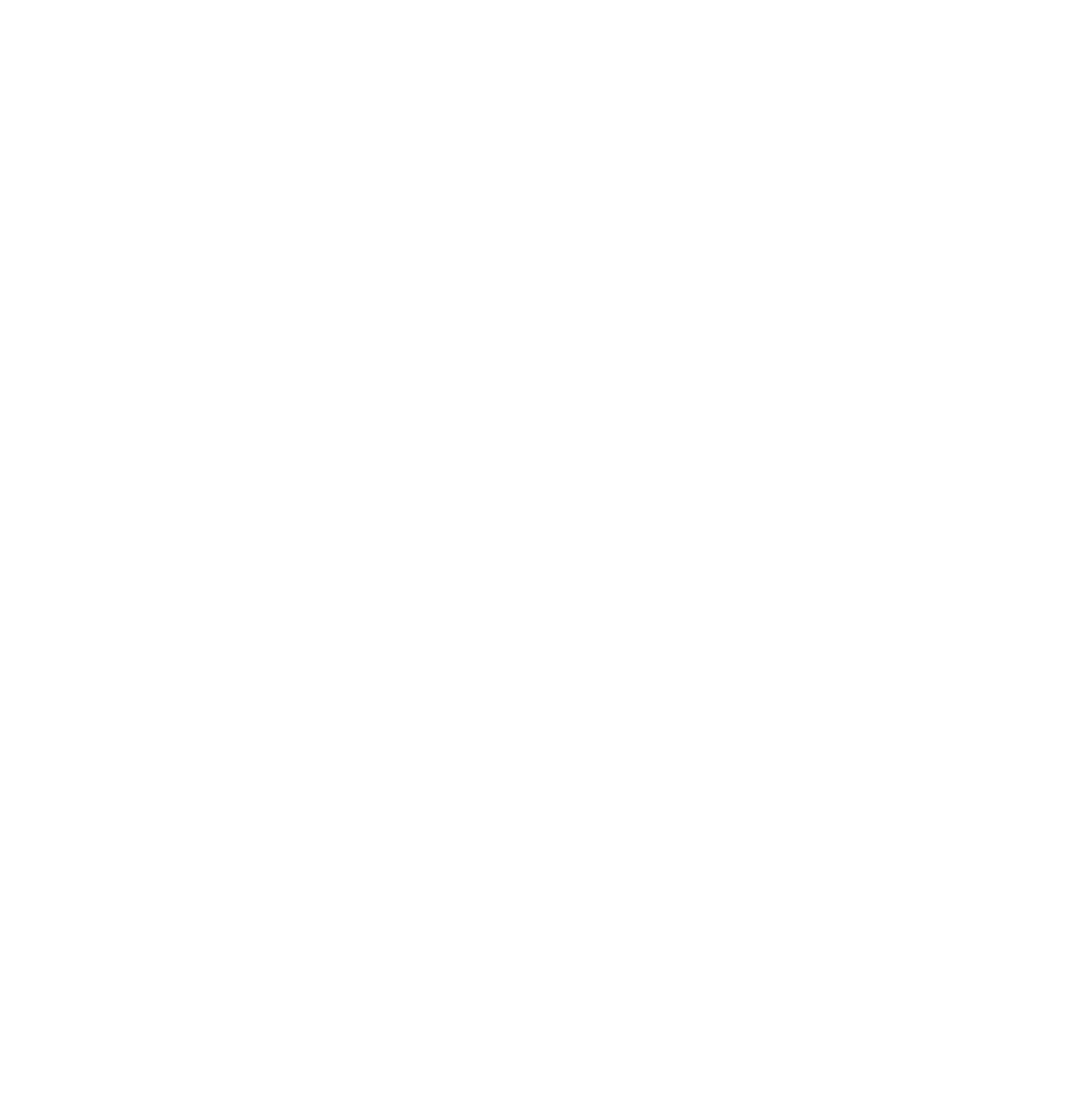
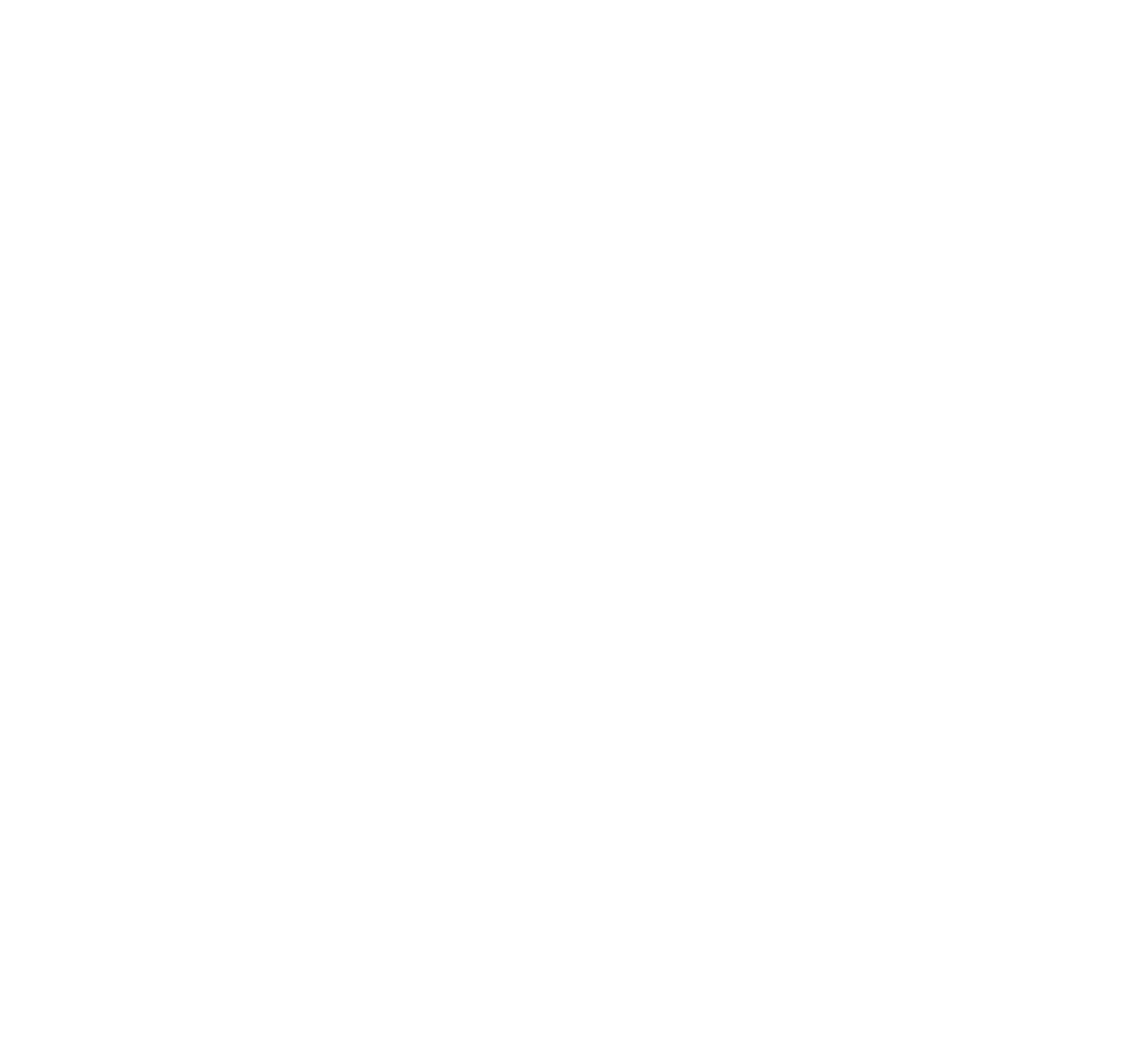
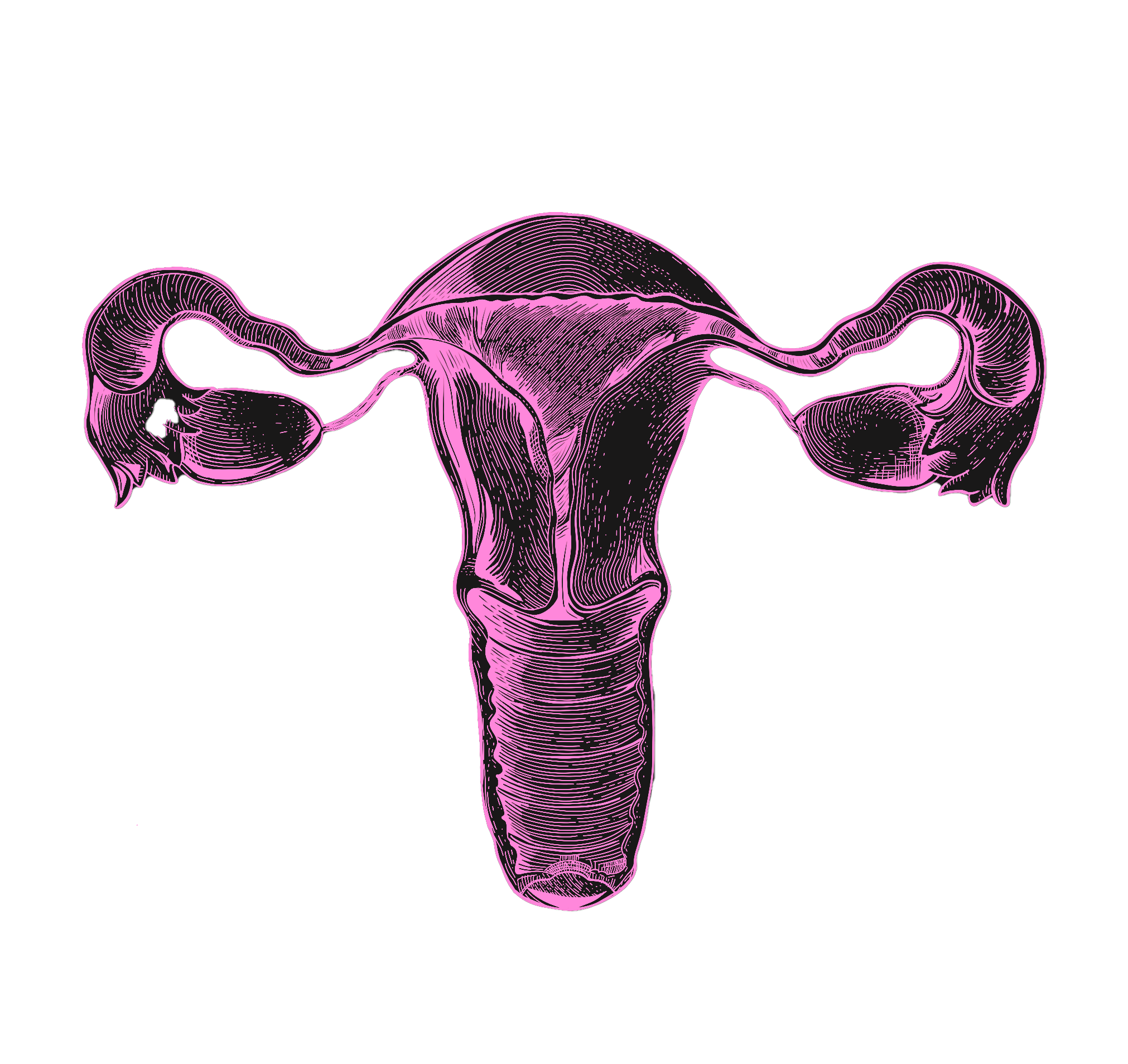
to U.S. GDP
Net Present Value
or gained by 2050
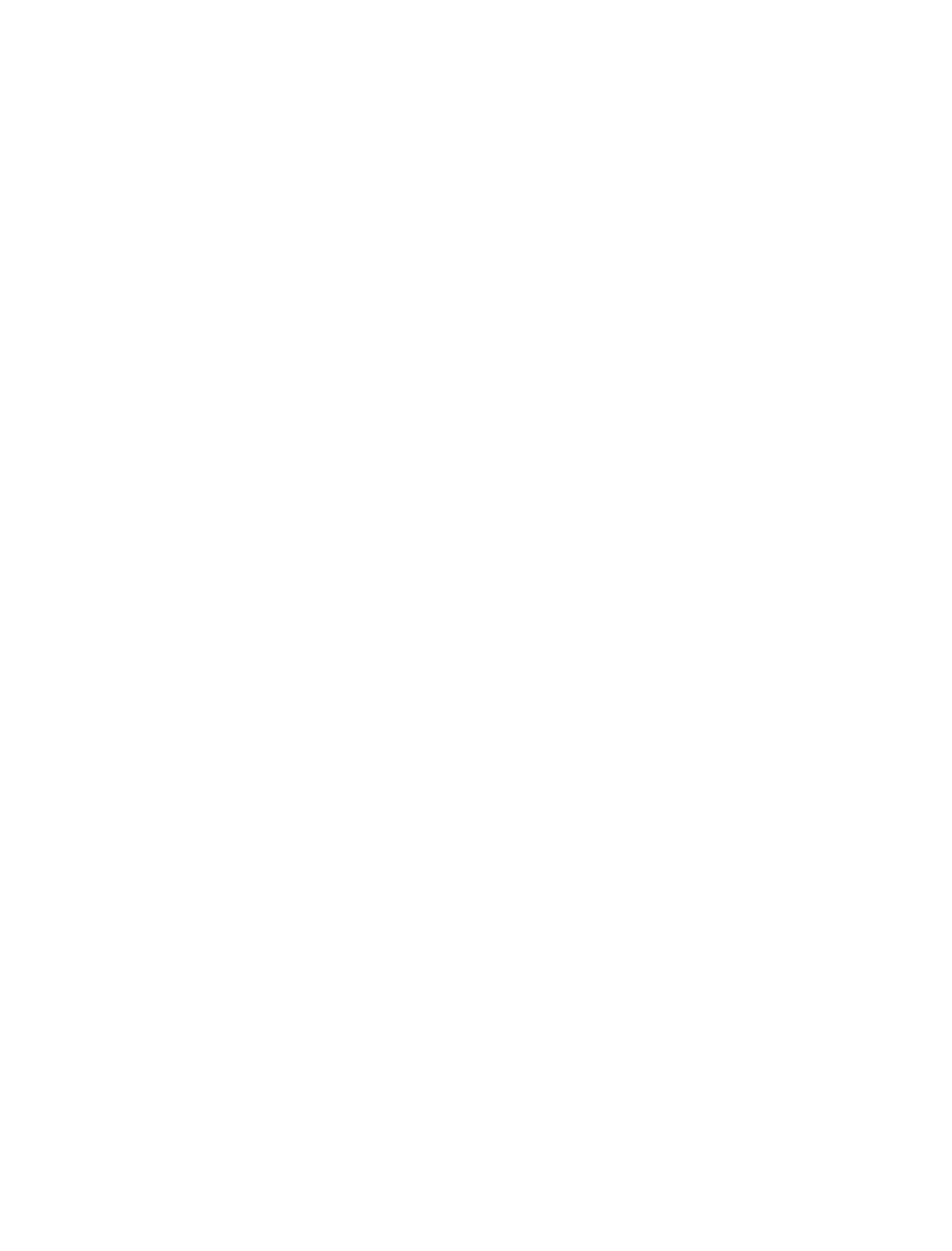
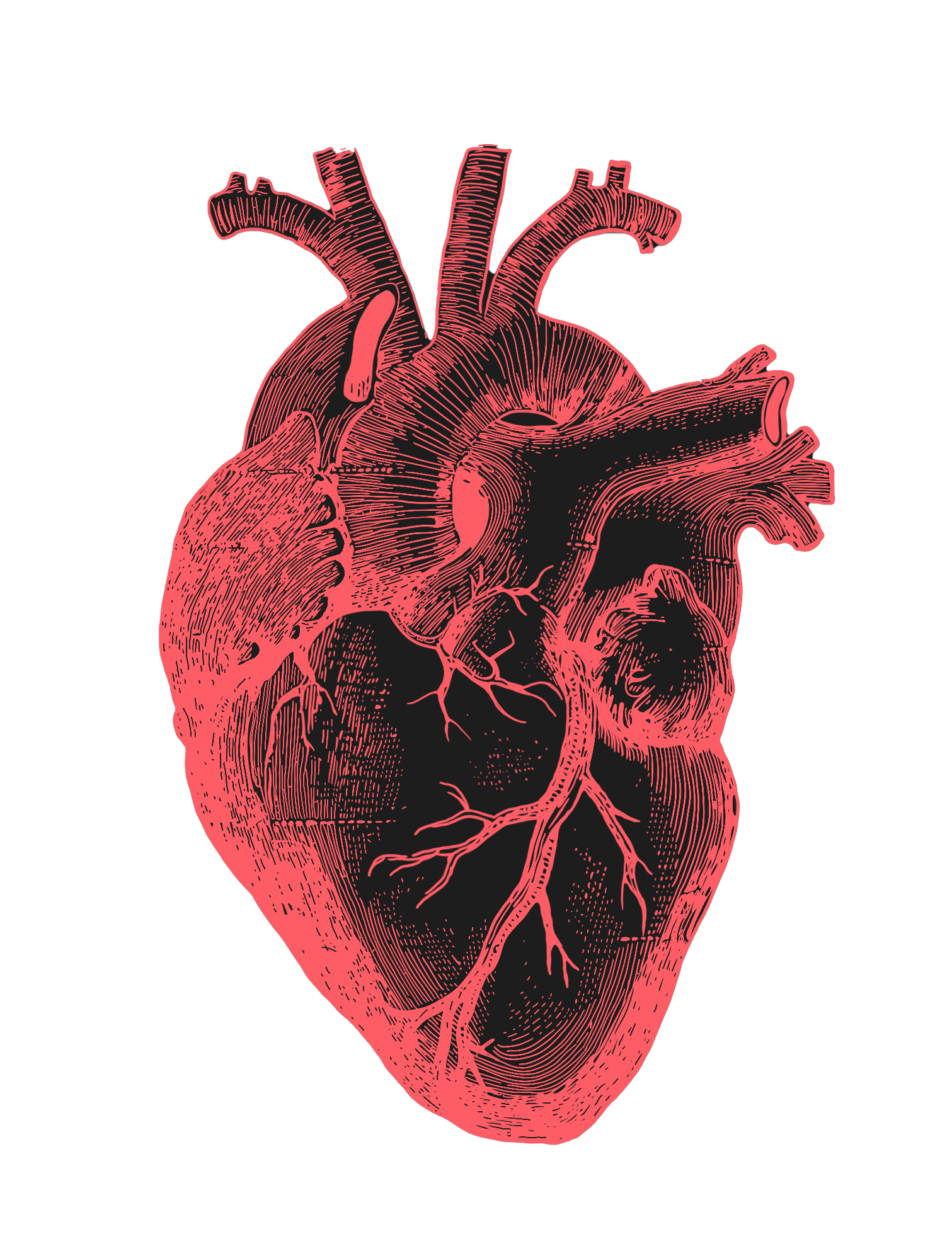
to U.S. GDP
Net Present Value
or gained by 2050
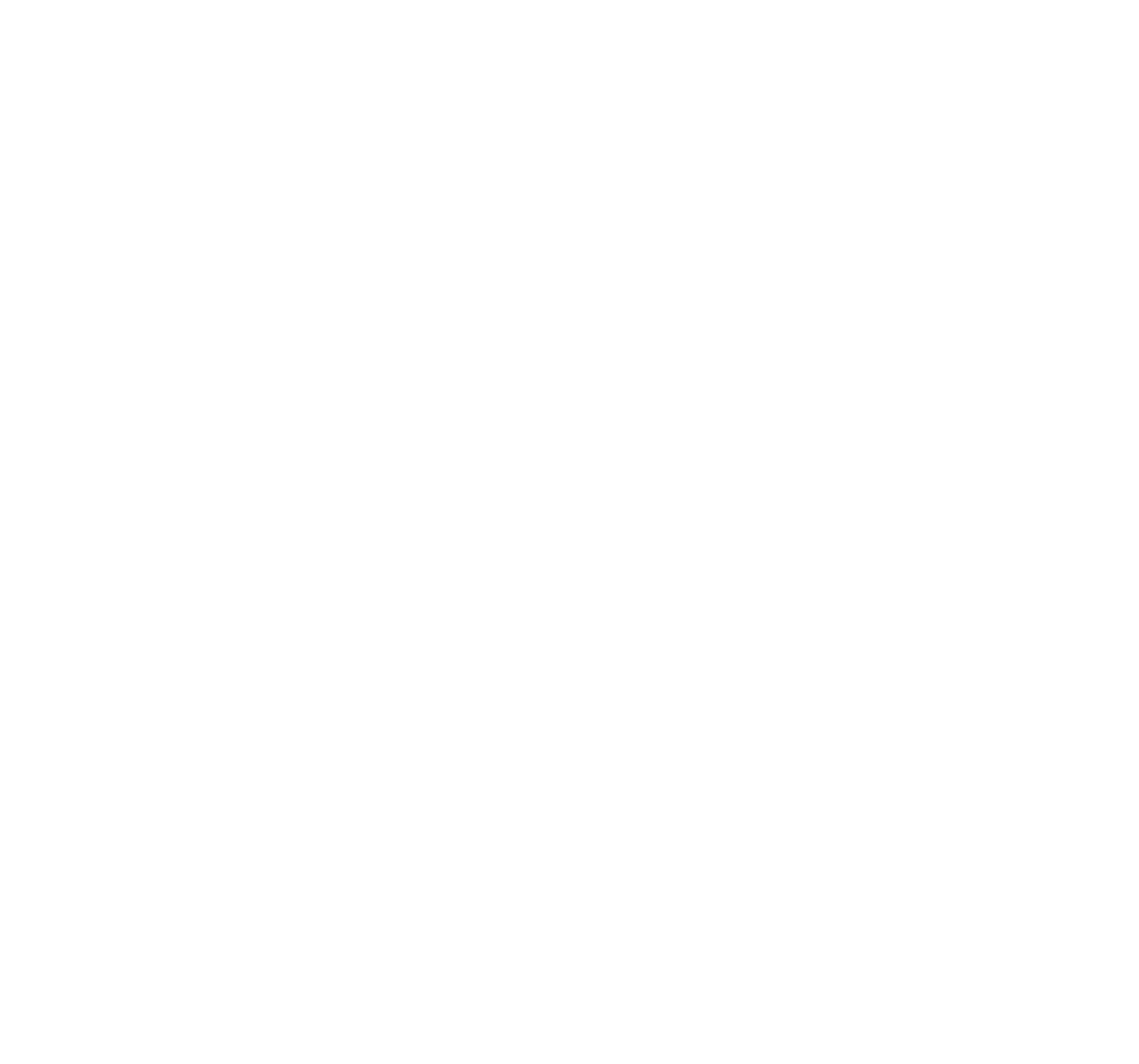
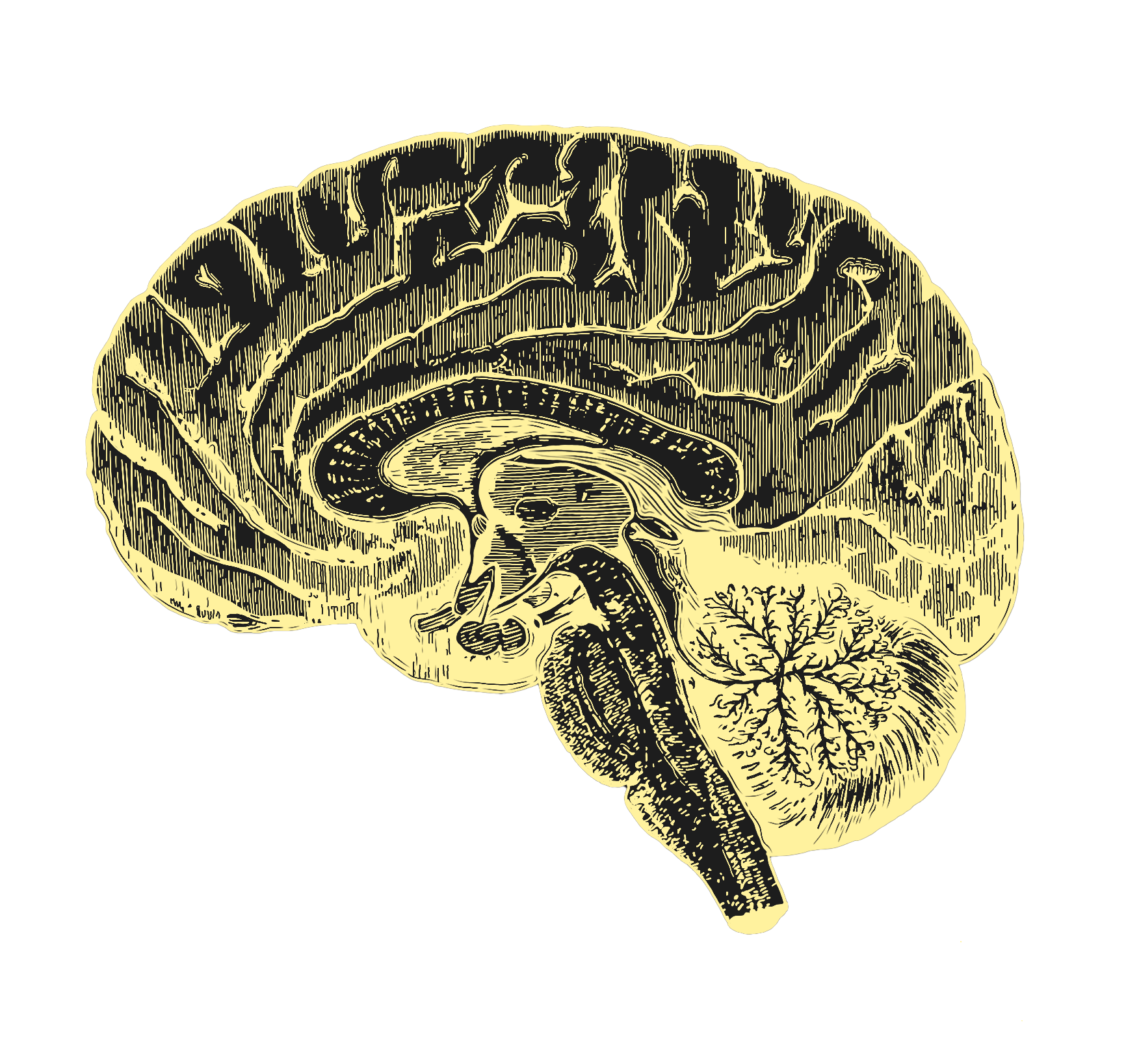
to U.S. GDP
Net Present Value
or gained by 2050
R&D opportunities in aging biology
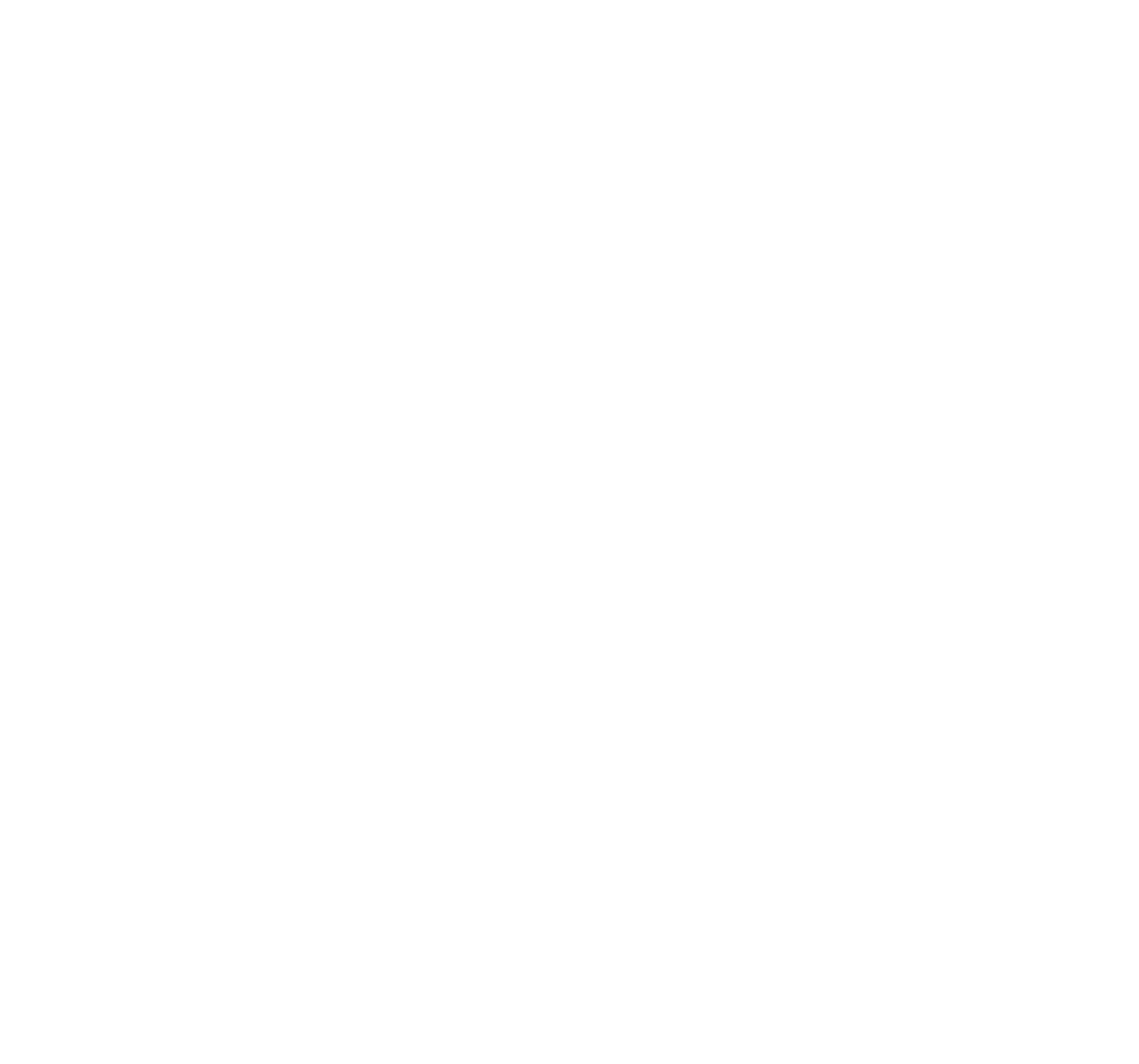
(Net Present Value over decades)
(by 2050)
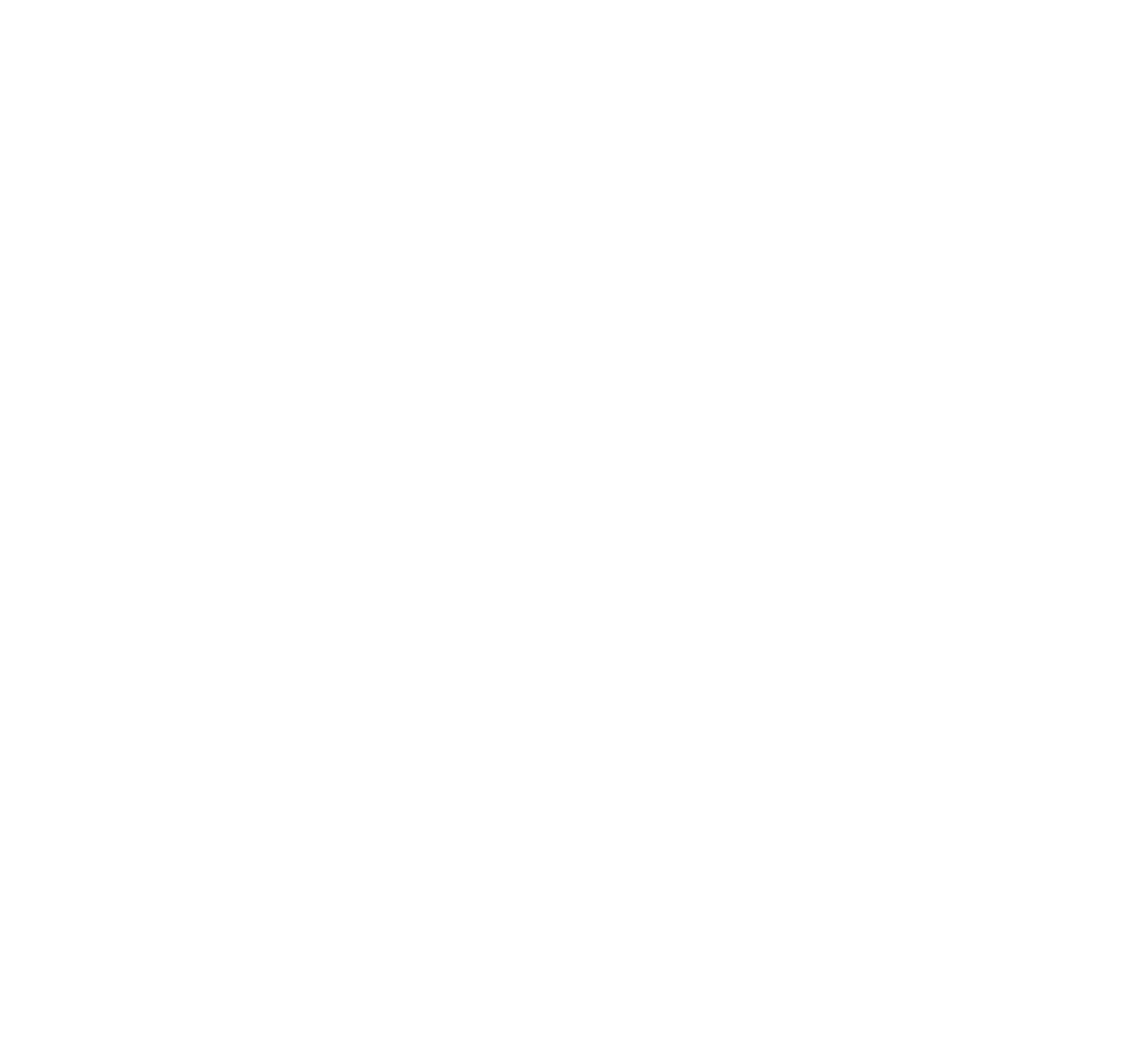
(Net Present Value over decades)
(by 2050)
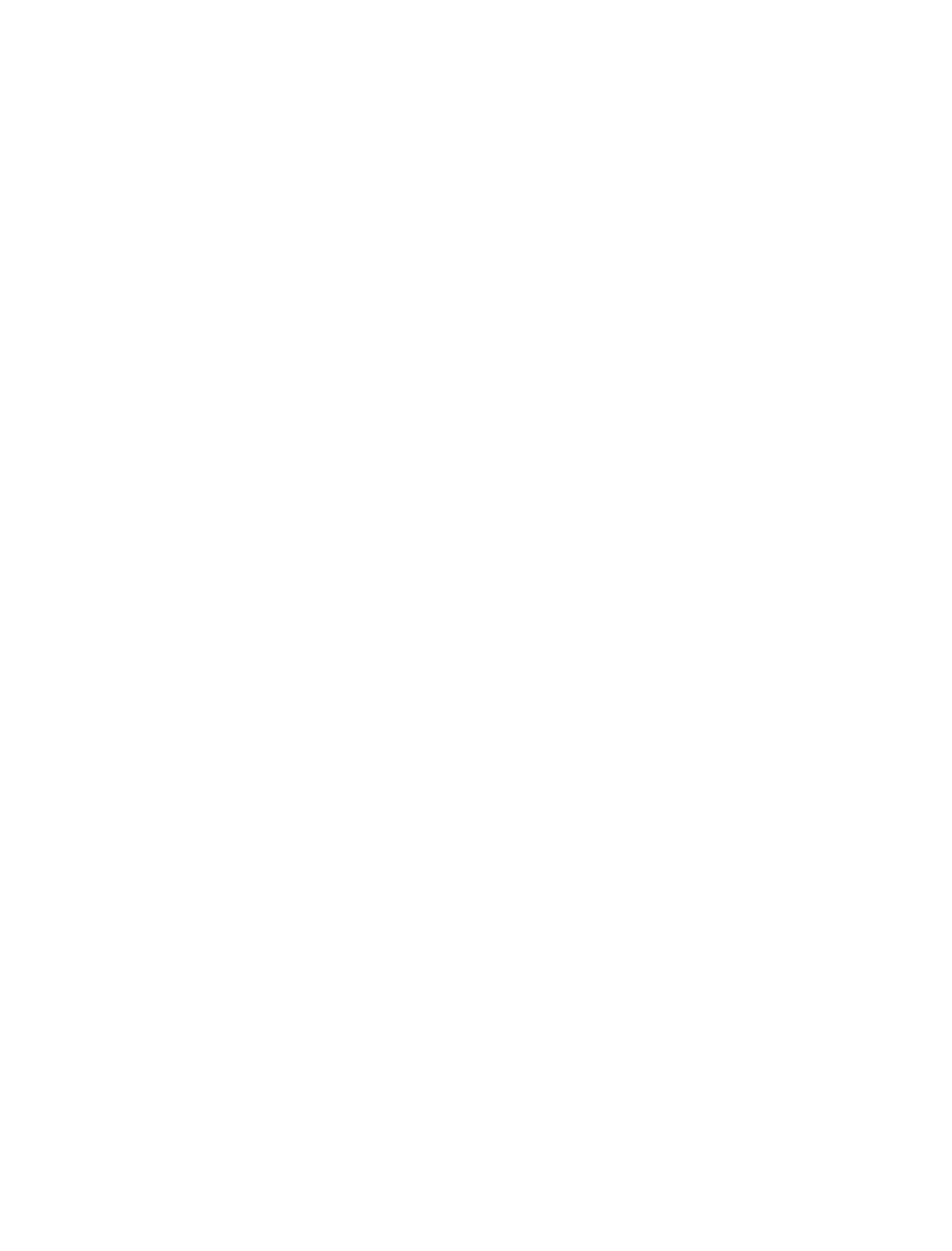
(Net Present Value over decades)
(by 2050)
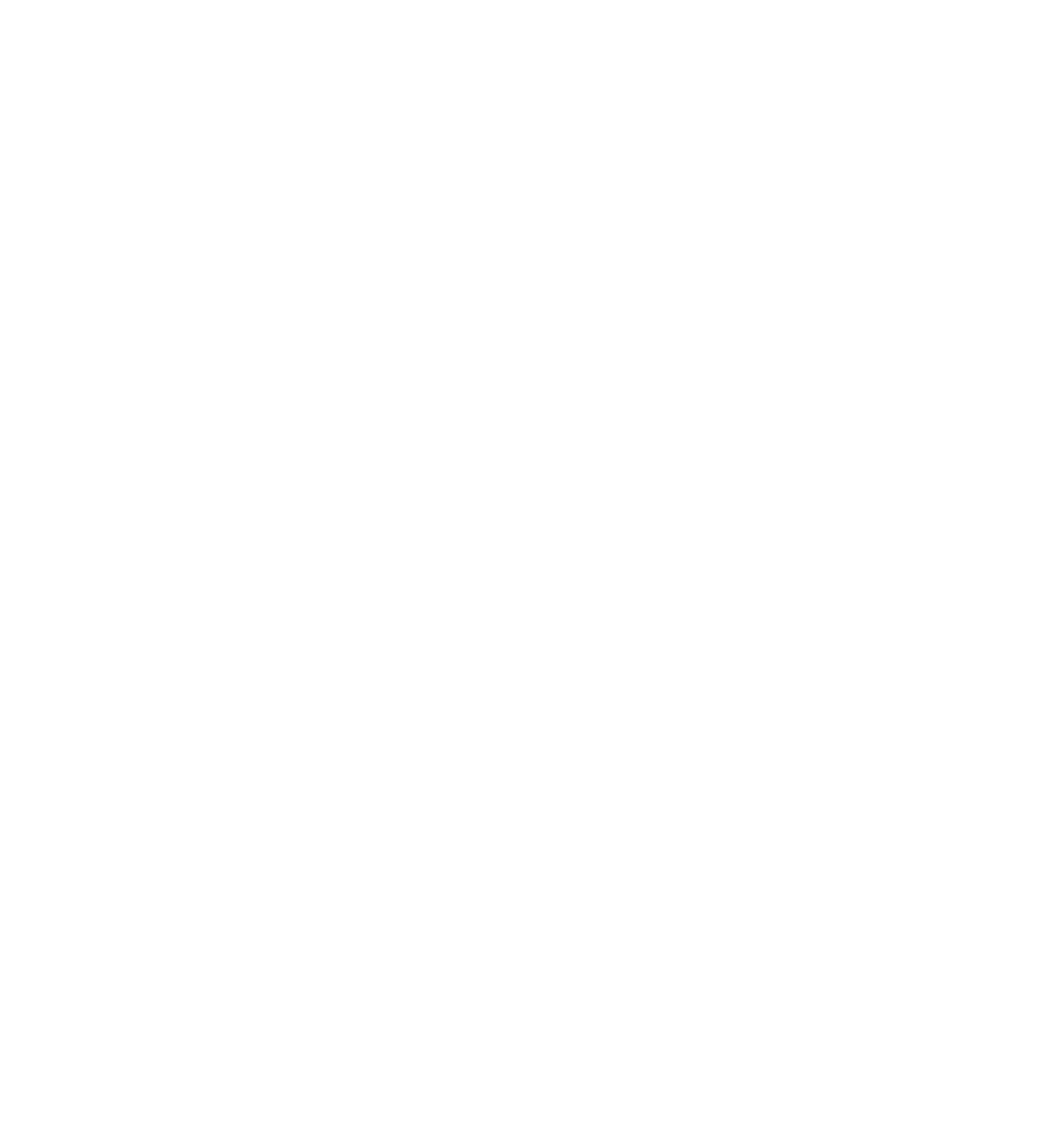
(Net Present Value over decades)
(by 2050)
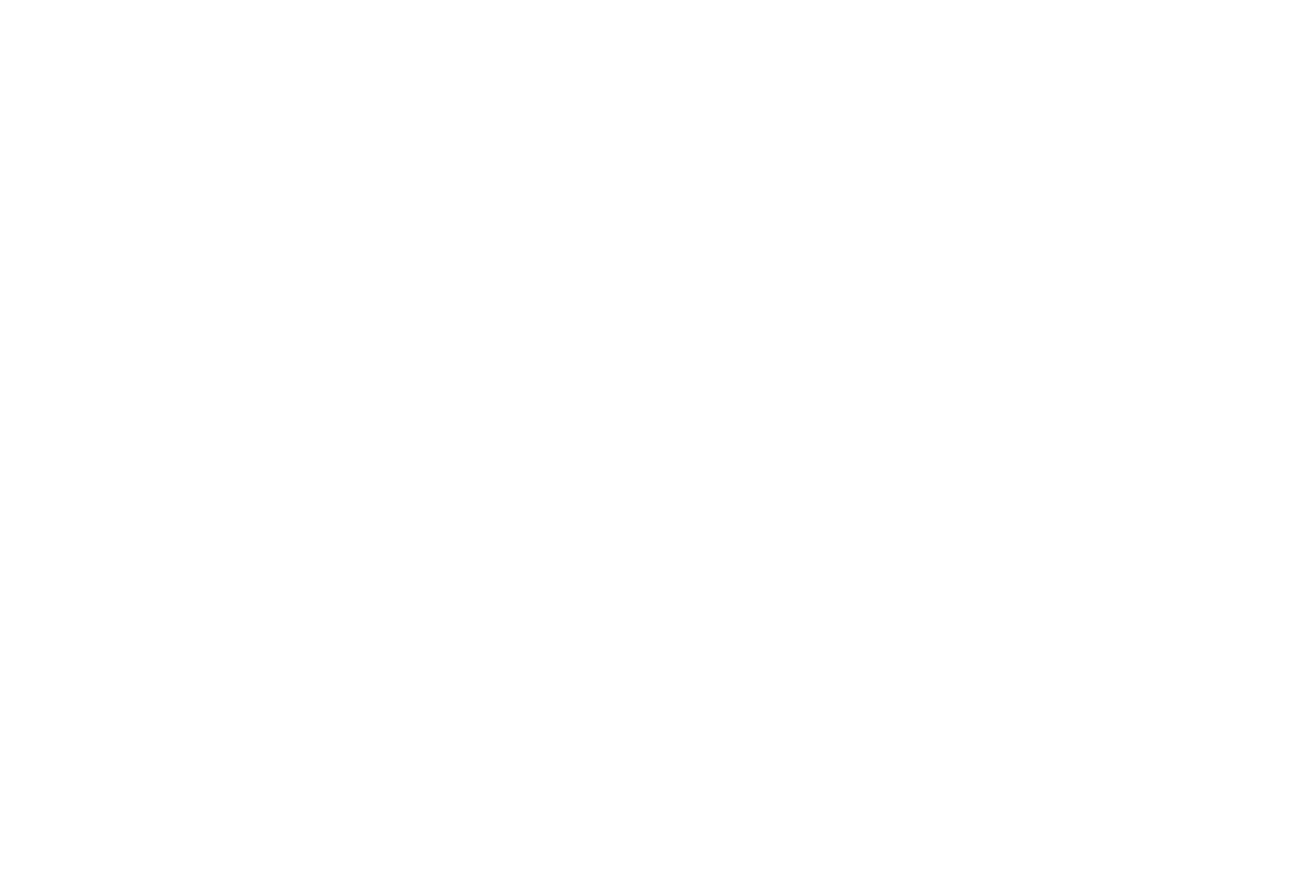
(Net Present Value over decades)
(by 2050)
It’s a forgotten truth that — more than oil, gold, or data — working-age adults are the most valuable resource of our time.
Scientists, nurses, thinkers, bakers, engineers and builders make up the backbone of our economies. The United States remains the world’s most powerful country, in great part, because it attracts the world’s most talented and productive people. It is also the third most populous country in the world, behind only India and China. And for as long as robots and artificial intelligence fail to take over our jobs and engineer a welcome era of abundance, the ceiling of the world’s economies will remain largely fixed to the health and number of their working-age adults.
The world is aging, and more births would be a partial solution to the emerging shortage of working-age adults worldwide. Yet they would be no silver bullet. In the short term, higher fertility rates worsen dependency ratios (newborns don’t work, and they temporarily remove their parents from the workforce); in the long term, they leave the sky-high socio-economic costs of aging unchanged. In a rational universe, healthy aging would be a top priority of governments, foundations, families, and investors worldwide. Yet many market failures and misaligned incentives stand in the way of private and public investments into extending healthy life.
The diseases of aging still begin to show up at nearly the same age as they did in 300 BC — and they follow a predictable trajectory throughout our lifespan.
Age is the primary risk factor for the diseases of aging. The median age of a cancer diagnosis is 66; the first heart attack, 65; and a dementia diagnosis, 83. Biological aging is also a neglected factor in global pandemics and even rare diseases like progeria or childhood cancers, where patients experience a form of accelerated aging. This explains why the value of investing in research on aging biology is high even compared to other excellent investments, since most severe conditions are downstream of biological aging. Eliminating all cancers, for instance, would add between 2 and 3 years to life expectancy. But since the median age of a cancer diagnosis is 66, the same patients would anyway soon be diagnosed with another manifestation of aging — like Parkinson’s, hypertension, severe illness from an otherwise mild infection, or a broken rib.
Scientific challenges help explain why we lack human-relevant results in aging biology. Yet they do not explain why, for instance, research on Alzheimer’s alone receives roughly 8 times more funding than research on the biology of aging, though we lack human-relevant results for safe Alzheimer’s drugs. Today, the United States spends a mere 0.54% of its National Institutes of Health research budget on the biology of aging. Due to a number of market failures and misaligned incentives, the vast majority of public and private funds go towards the treatment of late-stage conditions.
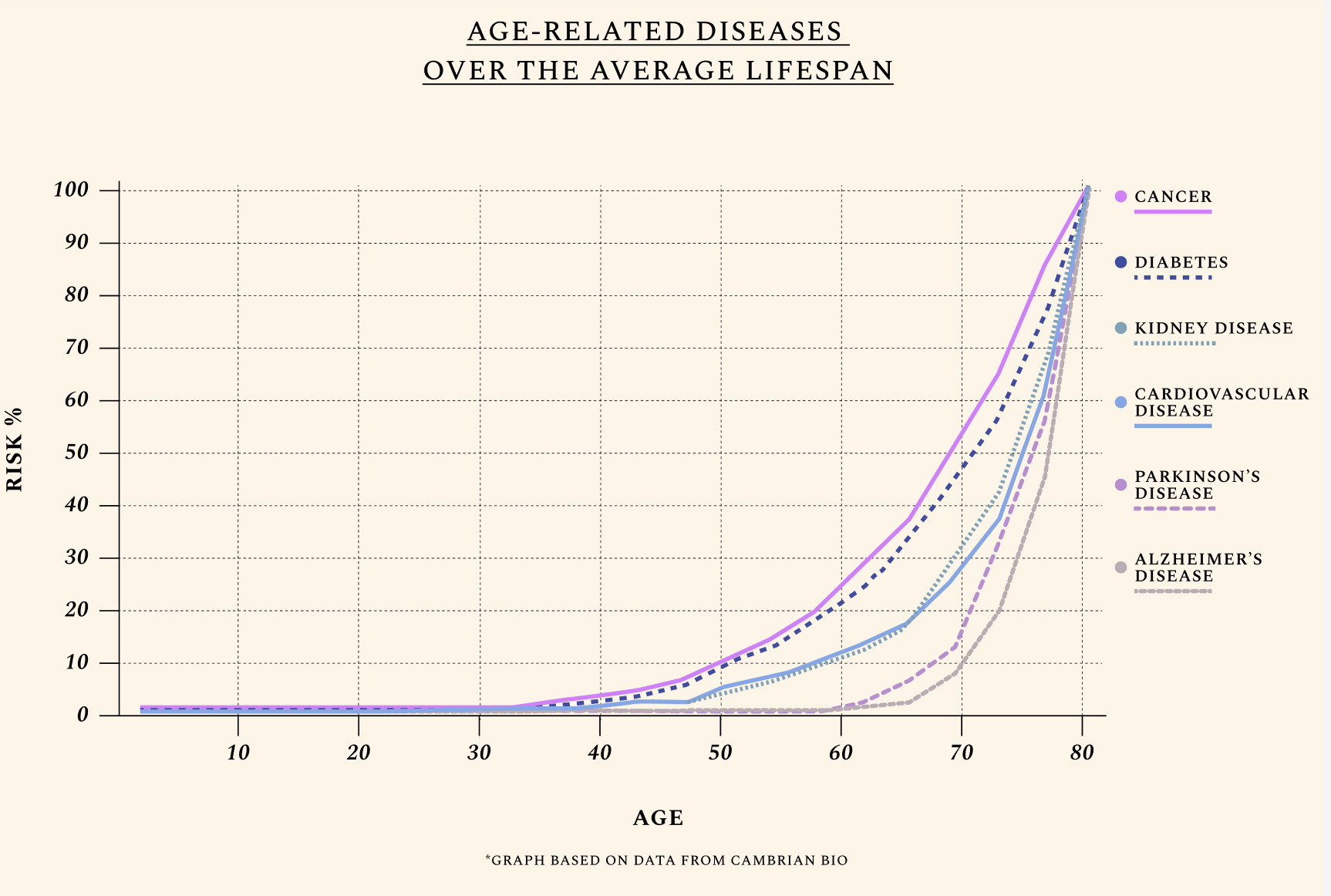
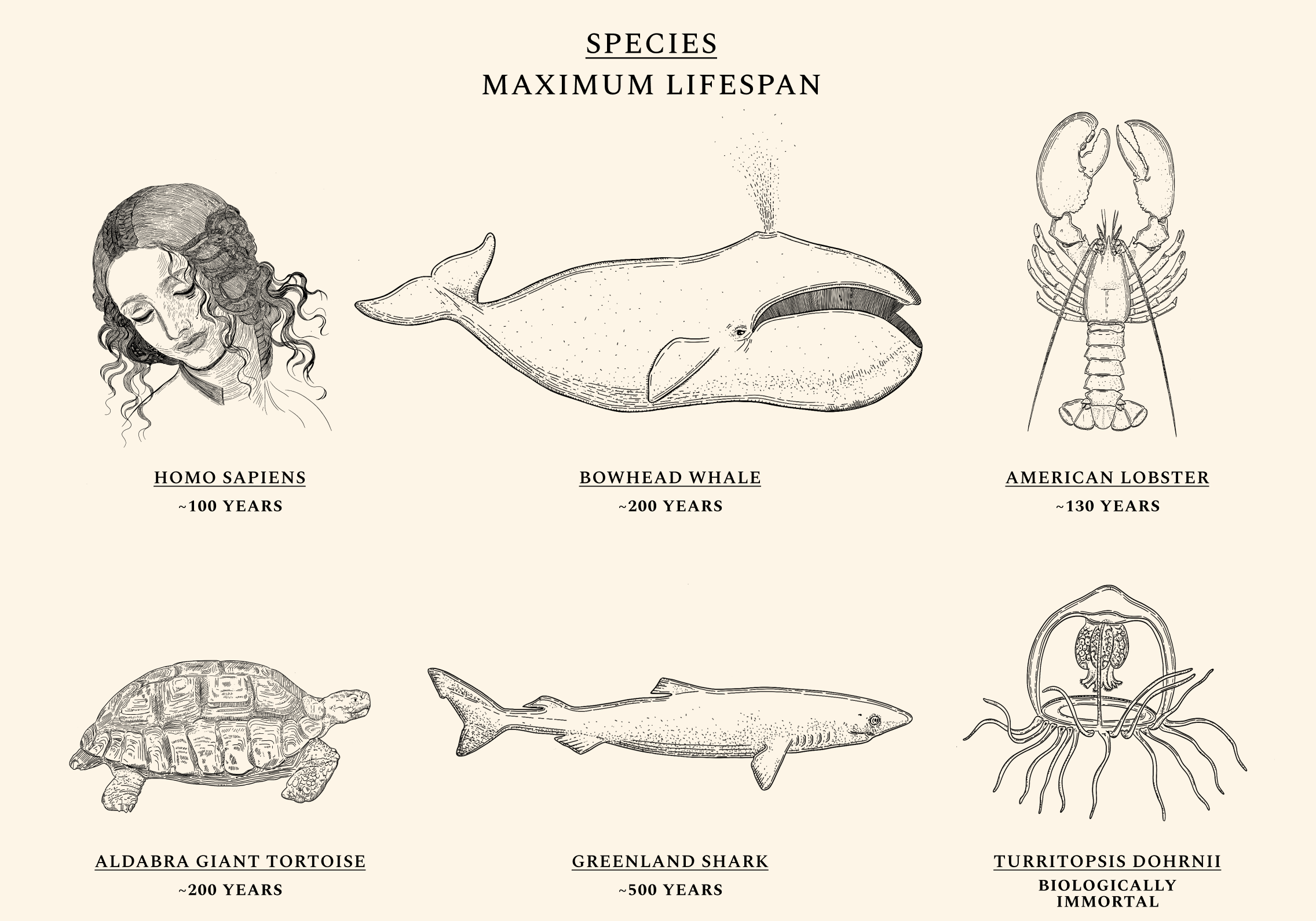
To start with, aging isn’t time.
Some species, like the Aldabra giant tortoise, are more likely to die the moment they are born than they are at age 90. Others, like the jellyfish Turritopsis Dohrnii, are often called "biologically immortal," which means that without extrinsic mortality rates (e.g. predators or infectious diseases) they would not necessarily die. Mammals like the bowhead whale and fish like Greenland sharks routinely live for centuries without developing chronic illnesses like cancers or Alzheimer’s, while the health of naked-mole rats appears not to decline at all over time. Humans exhibit a similar ability for health maintenance until our twenties. Throughout our lives, our cells constantly undergo mutations and alterations. But as our natural capacity for repair begins to wane, damage begins to irreversibly accumulate. The same type of molecular damage that might have been easily tended to at a young age begins to build up during our thirties and forties. The diseases of aging — like cancers, heart diseases, dementias, and diabetes — most often appear after decades of misrepairs.
It’s well established that some hallmarks of aging can be accelerated by habits like smoking, or by events like pregnancy and infection. Less obvious is the fact that biological aging can be slowed down and reversed. Indeed, some hallmarks of aging are temporarily reversed every day with diet, mental health practices, and exercise. Yet there is a low ceiling to what can be achieved with lifestyle interventions for primates with our DNA. Just as two-hundred-year-old tortoises won’t suddenly start aging poorly after several days of little movement on the beach, or after binge-eating the carcasses of other tortoises (a habit they sometimes indulge in), humans can’t buy cancer-free, two-hundred-year lives by self-starving or walking at giant-tortoise speed.
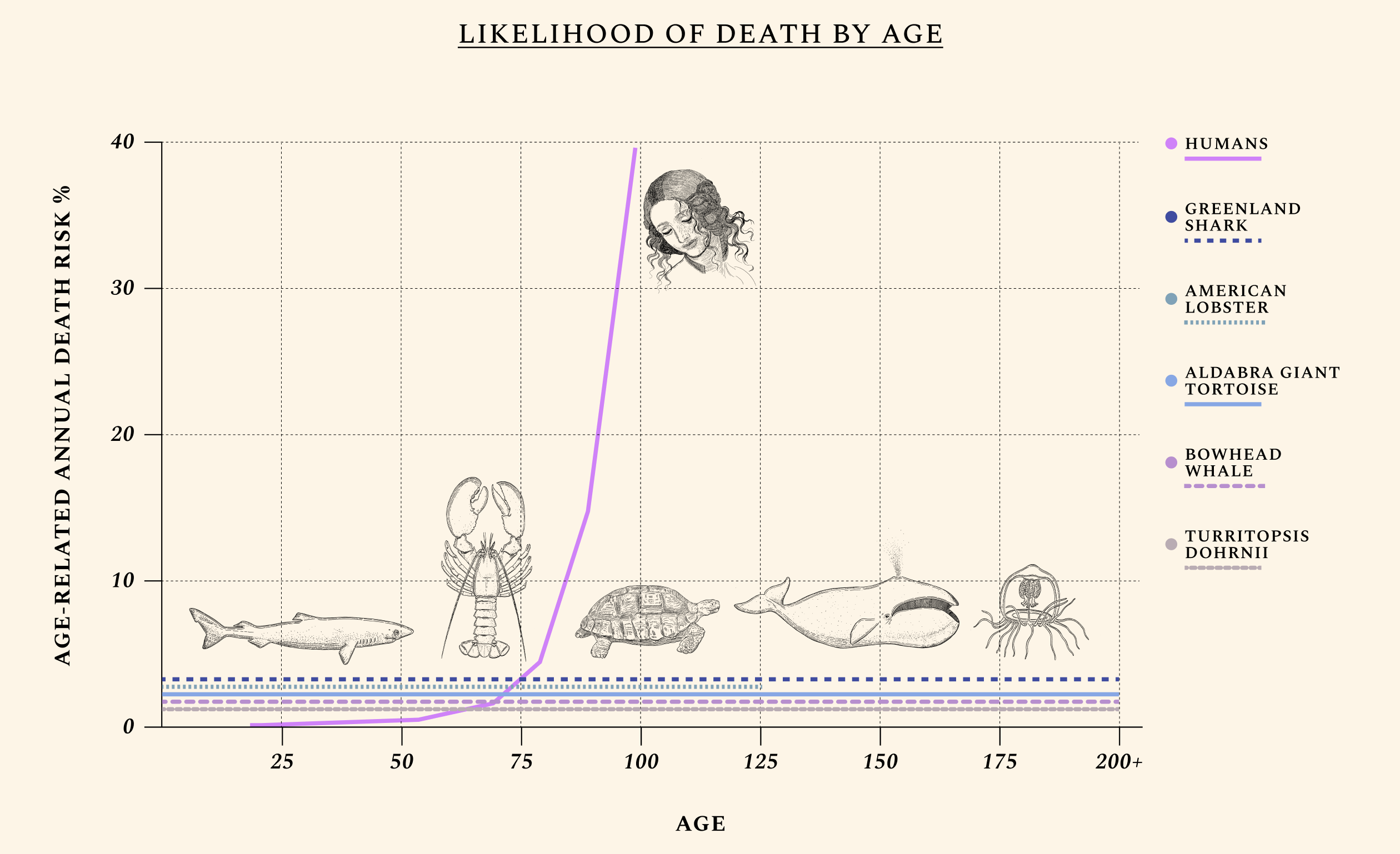
Aging isn’t one thing, equally manifested in all species — and this explains why scientists have such a hard time agreeing on just what it is.
We interviewed 102 scientists for this project. Some scientists understand aging as a “software design flaw”— a programmatic process that can be targeted epigenetically, by targeting gene expression rather than the genetic code itself. Others see it as a multifactorial set of processes whose causes demand more invasive solutions, like replacing tissues. Aging is a convenient word to describe the loss of function caused by a buildup in molecular damage over a species’ average lifespan. But different animals experience this loss differently (or not at all); different humans age at different paces (owing in part to different lifestyle choices, and in part to different genes); and different organs warrant different aging clocks. The ovaries, for instance, become geriatric some 40 years before the brain. For a discussion on why aging evolved in the first place, read the book, or peek here and here for two brief explanations.
What matters is that attempts at increasing health and lifespan have been successful in nearly every animal model studied so far. In a 1993 study, changing just one gene (daf-2, an insulin pathway humans share) in C. elegans worms doubled their lifespan. Changing one additional gene (rsks-1) resulted in a 500% lifespan increase, or the equivalent of a 400-year-old human in seemingly good health. In mammalian models, approaches like inhibiting mTOR activity or eliminating senescent cells often result in increased median survival rates. A short list of currently used therapeutics like rapamycin, metformin, GLP-1 agonists like Ozempic, and senolytics show signs of delaying multiple age-related diseases at once. Yet the risk-benefit profile of existing drugs remains unproven for most healthy humans, and the discovery of new and more effective drug targets should be prioritized.
You might think that in purely economic terms, human death is a net positive, since it mostly occurs to people dependent on costly social and medical care.
Yet the fact that over half of U.S. states recorded more deaths than births in 2022 can only be mourned. Healthy humans would impose fewer burdens on our medical and social systems. But even half-healthy humans are by far more productive (as well as happier and healthier) than dead ones; and more newborns are generally worth more to the economy, in the long run, than fewer.
The holy grail of aging science — and arguably of medicine — is to extend health at the same pace that it extends life. This would have substantial effects on the global economy, lowering the burden on caregivers, care receivers, and young populations who in part subsidize the medical and social care of older adults. Longer-lived and more productive humans would also lead to higher investments in human capital: more education, more spending, and more productive labor.
Economic growth is broadly driven by two factors: growth in the population, and growth in the amount each worker can produce within a given time. Labor productivity is the most direct way in which increases in healthspan impact the economy’s output. And in any century of recorded productivity so far, one pattern is clear: labor productivity rises in the first part of working life as experience and human capital accumulate, then declines later in life, as declines in physical and cognitive function overwhelm the increases in human capital. Improving the biology of aging implies slowing the rates of cognitive and physical decline later in life, which translates into slower decline in labor productivity.
People typically reach peak earnings as late as their experience has not been outpaced by cognitive decline.
This is shown by the graph below, which outlines how humans typically earn their highest income around age 55, followed by a steep decline caused primarily by the effects of biological aging. In the average lifetime, hourly earnings follow a near-perfect triangle shape, increasing as human capital goes up, then decreasing with age-related health decline. Improvements in the biology of aging are unique relative to other health investments in that they can extend one’s productive time at the apex of this triangle. This is what we mean when we discuss year shifts in productivity by age.
Higher fertility rates by age, by contrast, add to the number of triangles without meaningfully changing their shape. In other words, higher fertility by age creates more people without making existing ones more productive. But fertility rates would have little to do with biological aging if they did not also have the ability to at least marginally change the shape of the triangle – i.e., to increase productivity. In Future 2, we discuss how a better reproductive aging profile could lower the odds of disease and increase life expectancy.
Mortality rates by age can be counterintuitive. We all know that 60-year-olds are far likelier to die by any cause than 16-year-olds. What is counterintuitive is that life extension, even without a 1-1 improvement in healthspan, often increases GDP. The major way in which older adults become economically burdensome is through lower labor supply and increased medical and social costs. In our simulations, the underlying assumption is that older adults would be healthier and therefore more productive. This means that when discussing the biology of aging, shifts in mortality and productivity rates often go hand-in-hand. We assume no shifts in the age of retirement, and take into account only older adults who would voluntarily work past the age of 65, in good health. Interestingly, workers aged 65 and older are the fastest-growing labor group in the U.S. Today, many professionals (think physicians, teachers, politicians) refuse to retire despite their short cognitive healthspan. Others cannot afford to retire, and are forced to keep working even while suffering from age-related loss of function and dignity.
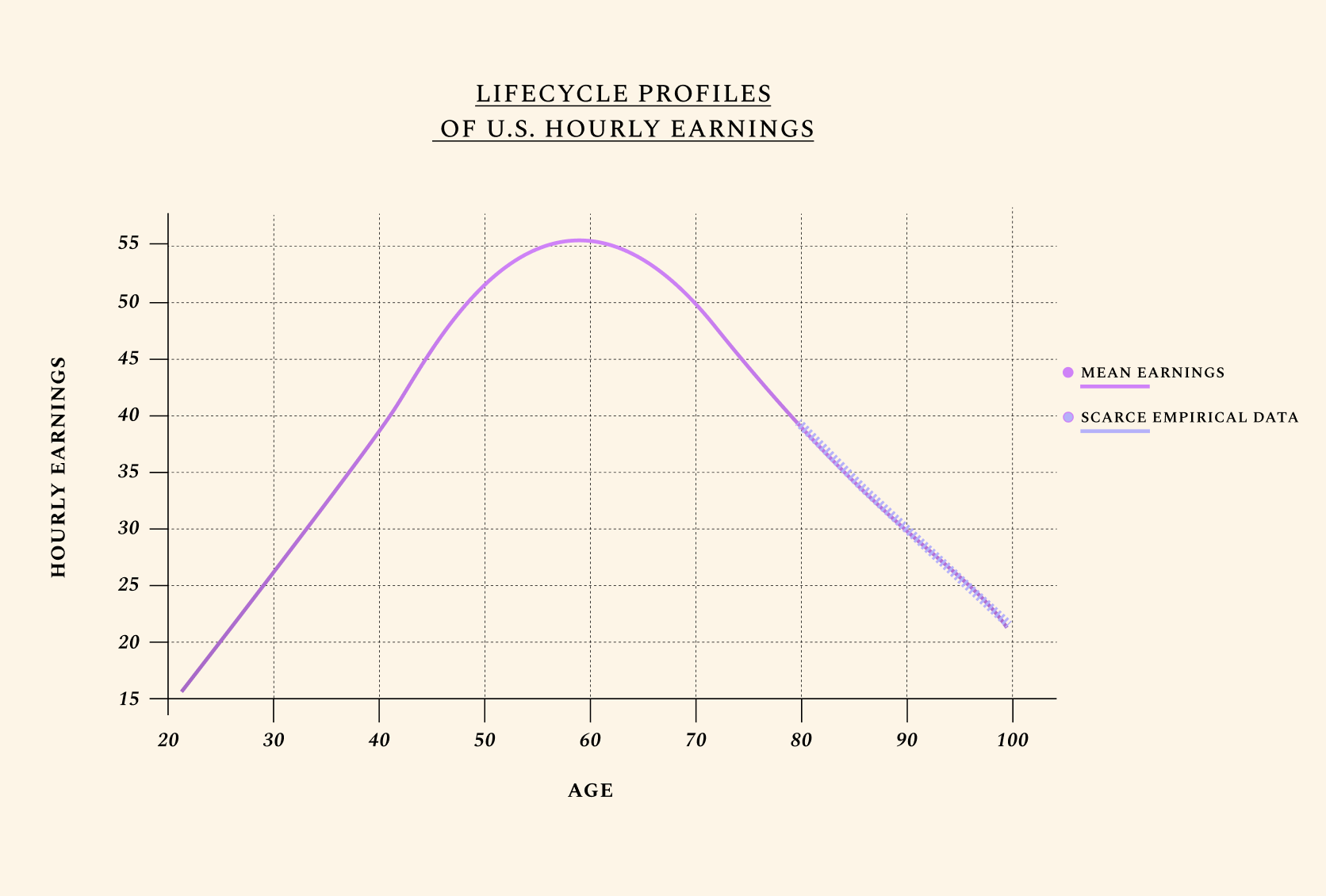
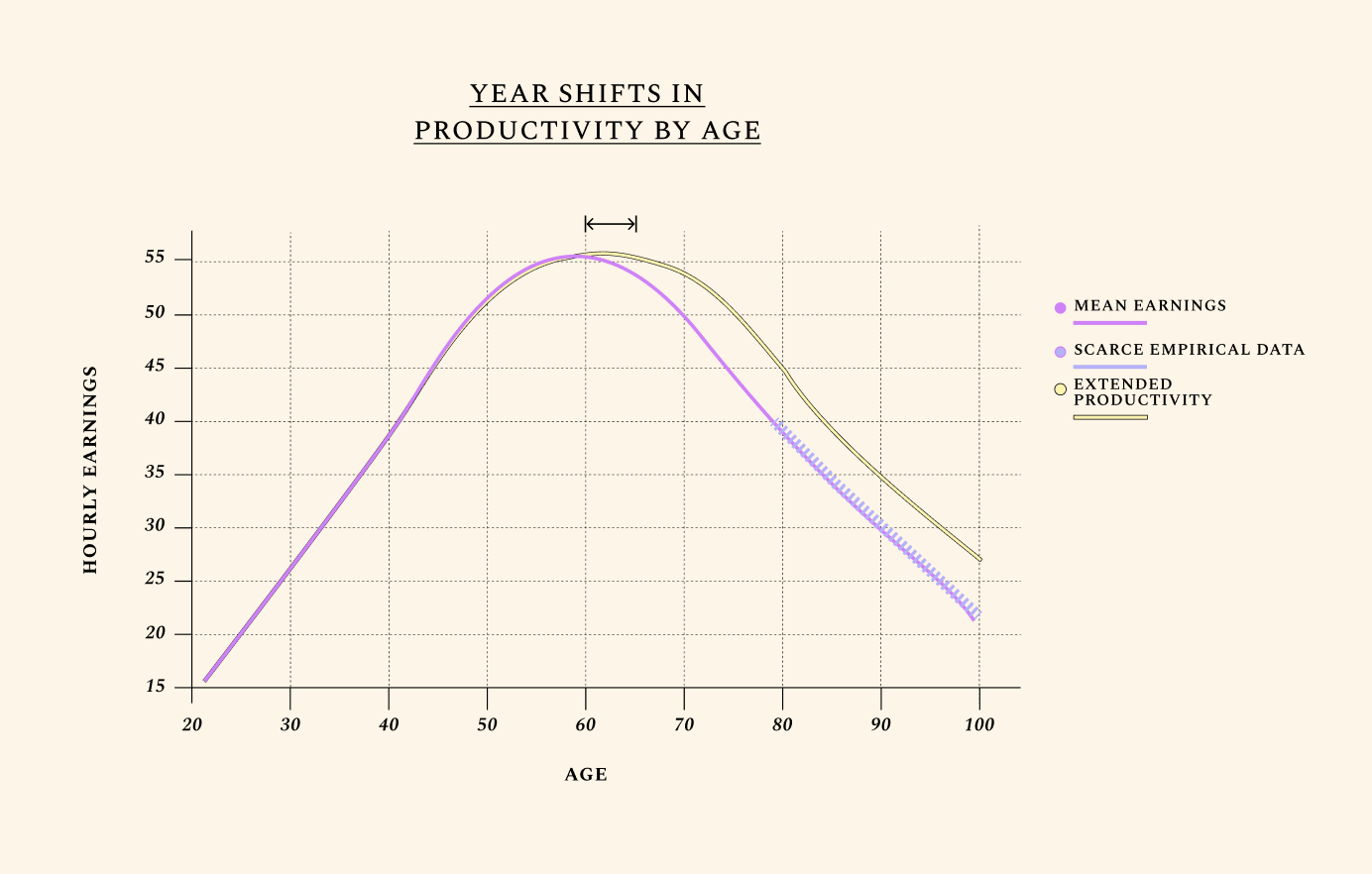
by just one year
At its most compelling, aging science would enable full health extension, so that the function of all organs would be rejuvenated somewhat simultaneously (just as the decline of our organs is somewhat simultaneous). This, however, is unlikely to happen in the immediate future. And if one organ among all others should be prioritized for rejuvenation, it’s the brain. How do we rejuvenate the brain? Probably not by waiting until it has noticeably failed.
Alzheimer’s research receives nearly $4 billion dollars annually from the United States government alone. Yet the existing focus on narrow approaches to the individual diseases of brain aging (like Parkinson’s and Alzheimer’s) has so far resulted in treatments whose risk-benefit profile remains discouraging. Research in this important area has traditionally neglected the root cause of most neurodegenerative diseases: namely, normal aging. Today, only 8% of the National Institute of Aging (NIA) budget is devoted to the biology of aging. Neurodegenerative diseases receive nearly 8 times more funding than fundamental research on aging biology. Yet brain function declines steadily with normal aging, with visible declines in cognitive performance at age 50. Therapeutics must be developed which prevent not just abnormal aging, but also this predictable decline.
Though a distinction is often made between “healthy” brain aging and age-related neurodegenerative diseases, the boundary between normal and abnormal neurodegeneration is blurred. At age 65, “less than 5% of the population has a clinical diagnosis of Alzheimer’s disease, but this number increases to more than 40% beyond age 85.”
Some animals — like songbirds, killifish, axolotls, lampreys, and planaria — naturally regenerate some parts of their brain. Others, like naked-mole rats, appear not to experience any cognitive decline. Animals with superior brain aging profiles remain understudied, and access to non-human primate brain samples, too, remains limited. Catalyzing these efforts isn’t commercially viable in the short term, and this creates a pressing rationale for public and philanthropic funds.
To simulate the effects of slowing brain aging on the U.S. economy, we assume these investments would pay off primarily by increasing the labor productivity of older workers and, to a lesser extent, decreasing mortality rates with age. We arrive at these values by computing two basic assumptions: that roughly 70% of all labor productivity is brain-related, while roughly 20% of all deaths can be attributed to the aging brain.(See 'Explanation' icon by simulation tool.) Readers are encouraged to input their own assumptions on the role of brain aging in mortality, productivity, and even fertility rates. (There is an unclear, but increasingly studied relationship between reproductive and brain aging, since the brain helps regulate fertility hormones.)
The attentive reader may notice that beyond a certain point, improvements in physical health or other non-cognitive functions have diminishing returns on productivity. A 1-year shift backwards in brain aging adds nearly as much to GDP as a 1-year shift in general biological aging in the near term, because returns from improving the aging profile of other organs (e.g. kidneys or ovaries) are not immediate, and sometimes even reduce GDP temporarily. Improving the cognitive healthspan translates into significant and immediate productivity gains. (+$555 in GDP per capita terms).
by just one year
Evolution optimizes for reproductive health, when possible.
From this, it follows that menopause is a relative rarity in the animal kingdom. Animals that experience no decline in fertility throughout their lives — like the naked-mole rat — are on one extreme end of the spectrum. Homo Sapiens is on the other extreme end. By some measures, humans are one of only five species in the animal kingdom who undergo menopause. American Lobsters, for instance, become stronger and more fertile with age, and not seldom live to be 100 in good overall health. Most non-human primates also continue to reproduce well into advanced age. In humans, however, the ovaries are the first organ to age — and men’s reproductive healthspan, too, declines more quickly than most assume. This short fertility window (pictured on the right for women) is responsible for several physical and mental health issues. In smaller part, it also contributes to the emerging shortage of working-age adults worldwide.
Not all couples would choose to have children in their 40s if they could healthily do so. Yet at least 11% of females seek fertility treatments at some point in their lives, mostly due to age-related infertility. In Australia, for instance, assisted reproductive technologies have so far been documented to increase total birth rates by 5% — a small but meaningful amount.
This research area would do more than just increase birth rates. The health and social costs of ovarian aging are impossible to simulate fully in dollars, but even a narrow measure of its effects on GDP produce staggering results. It’s well established that women who undergo menopause later live longer and suffer from fewer age-related diseases like dementia and osteoporosis. It’s no wonder, as reproductive aging scientist Jennifer Garrison notes, that today, when young women — typically under 40 — are diagnosed with menopause, “the medical community treats [it] as incredibly serious.” Yet the predictable decline of ovarian function with age (which coincides with a number of deadly diseases) after age 50 is understood as natural, inevitable, and not in need of treatment.
What if we could slow reproductive aging by just 1 year?
Given the currently short human healthspan, undergoing a geriatric pregnancy — understood as any pregnancy above age 34.3 for women — is both costly and dangerous. We do not yet understand what causes the female fertility window to last roughly 30 years less than the male window; why the reproductive span correlates with lifespan; or how aging ovaries or testes influence overall aging. What is certain — given precedents in animal models like American lobsters — is that a short fertility window is not a prerequisite for late-life health.
Therapies which extend the reproductive healthspan could positively affect the health profile of older adults, and over several decades, increase the number of working-age adults at any given time. Little data exists on the relationship between reproductive aging and productivity. It’s possible that the major gains from improving reproductive aging may come not from increased fertility rates, but from the overall healthspan gains accompanying delays in menopause and the prevention of age-related miscarriages and maternal mortality rates. This would mean increases in GDP per capita as well. Still, our baseline assumptions show that even modest increases in fertility rates — on the order of about 1% — can produce extraordinary effects in the long run.
The births enabled by a 1-year shift in fertility rates with no improvements in productivity or mortality rates would detract roughly $3 billion per year from GDP in the near term. This is because newborns don’t work and because pregnancy and parenthood temporarily translate into lower labor supply. But for our baseline simulation, we consider small improvements in mortality and productivity rates, and arrive at a +$11 billion yearly gain to GDP in the short term. (+0.07 cents in GDP per capita terms.) Compared to investments in brain aging, where the average near-term return is $364 billion per year, investments in reproductive aging are roughly 30 times less valuable in the near term. In the long run, however, the returns from this research area are extraordinary, and can benefit existing humans by increasing the odds of new Einsteins or Karikos.
*Fertility rates are capped to represent realistic increases in birth rates as enabled by reproductive technologies like IVF.
If the idea of “replacing aging” sounds messy, it’s because it is. But it may be one we cannot afford to ignore. Moonshot approaches like vaccines for aging or partial reprogramming may result in exciting, but limited gains. To fully map out the biology of aging, we may need several decades of fundamental research. But even if aging cannot be fully understood in the near term, its parts can be replaced — as shown by the replacement of cells, organs, blood, and tissues since the 1950’s.
The decline of organ function is a common symptom of aging, and many people need their organs replaced as they age. In the near future, it’s unlikely that a single therapeutic will slow down the biological age of all organs, cells, and tissues in the human body at once. Instead, a combination of biotechnologies will likely be needed. And the good news is that the commercial incentives for replacement are somewhat aligned to social needs: there is no shortage of companies attempting to engineer organs, cells, or tissues. CAR-T cell therapies, for instance, are a form of immune system replacement; organ transplants are a way of replacing malfunctioning parts; and hormone-replacement therapies (like insulin) are often used to treat late-stage diseases.
In a future where the horrific organ shortages of today can be overcome, seemingly healthy patients, having accumulated non-trivial age-related molecular damage, may begin to have their cells and tissues gradually replaced if the benefits of doing so outweigh the risks. Replacing different body parts — large and small — is not just safely done within the context of today’s medicine; it may be necessary to holistically improve human aging in the coming decade.
A simulation of organ abundance for still-healthy humans may have been more consistent with this project’s arguments — and this will ideally be the far-future result of investments in this R&D area. Yet given the horrific organ shortages of today, we chose to simulate the effects of first meeting this unmet demand — not least because once it is met, the same biotechnologies can be used to supply seemingly healthy humans, in prevention rather than late-stage treatment of organ or body-part failure.
To see our assumptions for this simulation in detail, see the book. Briefly, we focused on a decrease in mortality rates only, since many transplant patients will live longer but not necessarily in better health. It is very likely, however, that advancements in this area will also result in increases in productivity in the long run. (Otherwise, they would not be improving biological aging.)
We refer to a Nature paper by Giwa et al. to consider two possibilities: a 2x and a 4x increase in life-saving organ transplants. The first (2x) results in a 0.22-year decrease in mortality rates by age for all U.S. adults aged 40 and older. The second (4x) leads to a 0.44-year decrease in mortality rates by age. These effects would be mostly driven by a greater number of working-age adults alive at any given time, even if these adults would not enjoy 100% perfect health. The reader is also free to assume that organ, cell, and tissue transplant techniques will be refined in the coming decades to also extend healthspan and increase productivity rates by age.
If the reader considers only what is likely in the near term — namely, life-saving organ transplants for terminally ill patients — the impact of a 4x increase in organ supply on GDP per capita would be negative, at -$317. This is consistent with the existing sick-care system. If, however, the reader assumes this R&D area could eventually prevent age-related decline in still-healthy adults (for instance through cell engineering), the impact on GDP per capita would be overwhelmingly positive.
We can measure & marginally slow aging
How do we measure aging? In 2024, the depressing answer is nobody knows.
Or at least not precisely. Apps designed to track one’s biological age, while commercially viable, are often misleading. In the early 1950s, we had no effective therapeutic for heart disease for somewhat the same reason: LDL cholesterol was not yet approved as a surrogate marker of heart health, which meant the effects of statins and other lipid-lowering drugs could not have been precisely measured in clinical trials. The aging field suffers from a similar measuring problem today. Birthday candles measure our chronological age, but there’s no universally accepted way to measure how old we are biologically.
This means that even if an existing drug compound can improve aging with minimal side effects, proving this remains difficult. Because trials also typically measure gains in single diseases, the aging field suffers from the undervaluing of existing therapeutics which may improve overall biological aging if administered earlier. Here, we consider the value of two drug classes: existing, but imperfect therapeutics that may marginally slow aging — but have not yet been tested — and second-generation therapeutics that could be developed to delay aging in healthy, working-age adults who have no preexisting condition but will still go on to suffer from one of the diseases of aging. Then, we consider the delta in terms of lives saved and GDP increases between the current (first-generation) way of treating late-stage diseases versus early prevention (second-generation). This is one way of measuring the economic value of measuring aging. This delta adds up to $469B/y to U.S. GDP, adding up to roughly $20T in net present value in the long run, and over 1M extra lives saved.
Yet we note that even the adoption of existing therapeutics for aging as an endpoint could unlock extraordinary returns. Even given the assumption that only 11% of the population would benefit from this 1st-gen therapeutic, the TAME trial could generate a 430x return over 10 years in GDP growth alone.
The aging field is particularly vulnerable to the oft-cited problem that chocolate, if tested on dogs, would be deemed toxic.
No animal model captures the complex biology of human aging — and this means that for aging science to succeed, the advancement of breakthrough tools to test, measure, and model the biology of human aging may be critical. Another way to quantify the value of measuring aging is to consider the economic value of compressing the timeline of drug development. New technologies like organs-on-chips and virtual cells may help deliver on this. Organs-on-chips have the potential to mimic the structure and function of human organs in a microscale format, enabling clinical trials to measure the age of human organs with results perhaps more likely to translate to full-sized human bodies. For in vitro and in silico models to reproduce key aspects of aging biology, a better understanding of how human aging works (and what markers to include to represent it either virtually or in vitro) will be needed. In 2024, tools like organs-on-chips are only sometimes useful, and especially so in aging science, since it’s challenging to code “human aging” into them. Yet in the coming decade, a convergence of technologies could change this.
Previous studies estimate up to a 20% reduction in development time from using human-relevant methodologies like organs-on-chips or virtual cells in preclinical development. To simulate the economic effects of advancements in human-relevant methodologies (2nd-gen+), we consider the value of our 2nd-generation therapeutic simulation, boosted by a 20% reduction in development timeline. We narrowly measure these effects on one counterfactual trial; yet this technology could be applied horizontally to hundreds of trials.
This delta alone translates into a $18 billion yearly increase in U.S. GDP the short term, adding up to $1 trillion in the long run. This simulation is especially conservative because in the long run, these methodologies could not only reduce R&D costs and compress trial timelines, but also translate into therapeutics that safely improve human aging and prevent disease, as simulated in Future 5.
(or 60 the new 55!)
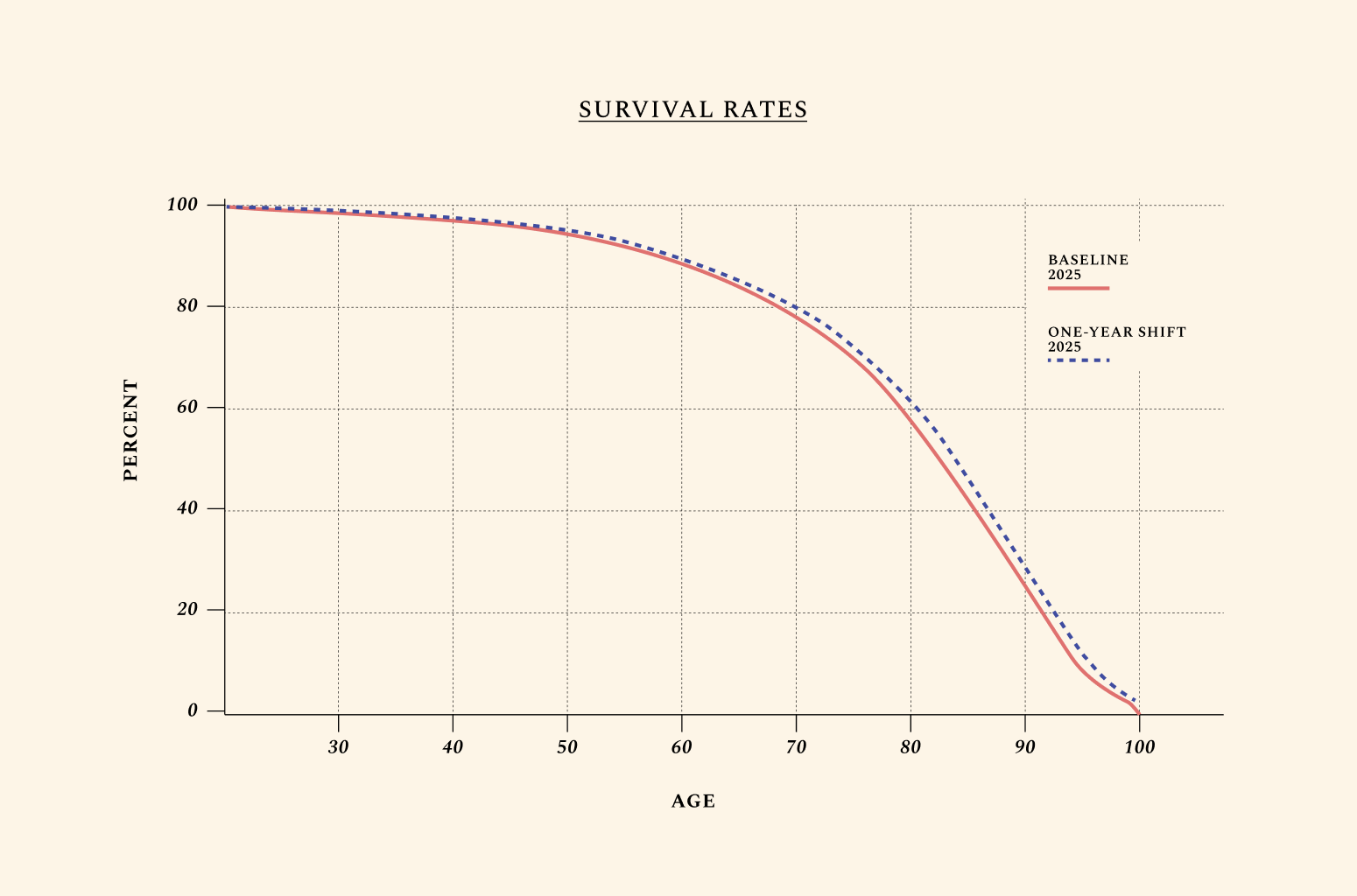
The holy grail of aging science — and arguably of medicine — is to extend health at roughly the same pace that it extends life. At its most compelling, aging science would unlock not just functional gains for discrete organs or tissues, but whole-body benefits. At their best, aging therapies would mean that not only the human reproductive window or the brain’s healthspan would be extended, but the onset of age-related conditions like osteoporosis, frailty, or Parkinson’s would also be delayed or reversed.
Treatments like GLP-1 agonists, for instance — even if they can successfully target multiple age-related conditions at once — have diminishing returns, since they were designed mostly for unhealthy patients. Improvements in biological aging, by contrast, may have less-diminishing returns, since the goal is prevention of age-related decline for still-healthy adults. Research suggests there may not be a limit to how many times the molecular damage that leads to the diseases of aging can be improved — but more research and translation are needed to produce these results in normally aging humans.
Here, we assume that the aging profile of different cells, tissues, and organs in the body could be therapeutically targeted, resulting in a 1-year improvement in biological aging. This is a very marginal improvement in the biology of aging. Yet its effects are large. Think of a world where 41 is the new 40, such that all adults over the age of 40 in the U.S. live, work, give birth, and die at the rates of adults 1 year younger. We call this 1-year shift “41 is the new 40,” but because we consider all adults over the age of 40 in the U.S, this shift would equally affect older adults, so that 61 would be the new 60, 71 the new 70, and so forth. Most scientists we interviewed are confident this marginal shift can be engineered with existing therapeutics that just haven’t yet been tested for biological aging in clinical trials.
This simulation is different from extending life expectancy by 1 year. Increases in life expectancy have been engineered many times before — for instance, by the introduction of refrigeration, with the ability to safely store food. Improvements in the biology of aging are different because they can simultaneously improve how we live, work, give birth, and die. In other words, improving the biology of aging means shifting healthy survival rates.
For more conservative results, the reader is free to assume that this 1-year shift backwards in biological aging would only affect older populations (e.g. 65+) already suffering from age-related diseases — in which case the returns would be smaller, in line with the existing sick-care system.
It is technically possible that a therapeutic may target the key causal node linked to aging across the human body. Cellular reprogramming, for instance, shows significant promise. Yet advancements in organ, cell, and tissue transplants may be needed to unlock a holistic improvement in biological aging, as simulated in this section. The human body is made up of complex adaptive systems comprising interacting networks of parts, and interventions like genome engineering for polygenic conditions may be decades away from safety and precision. This means a combination of interventions could be necessary to slow aging — including small-molecule drugs and refined technologies like tissue engineering.
The social net present value from our baseline simulation for this 1-year shift, adding up to $22.5 trillion over several decades, suggests roughly a 1.1% increase in U.S. GDP.
opportunities in aging biology
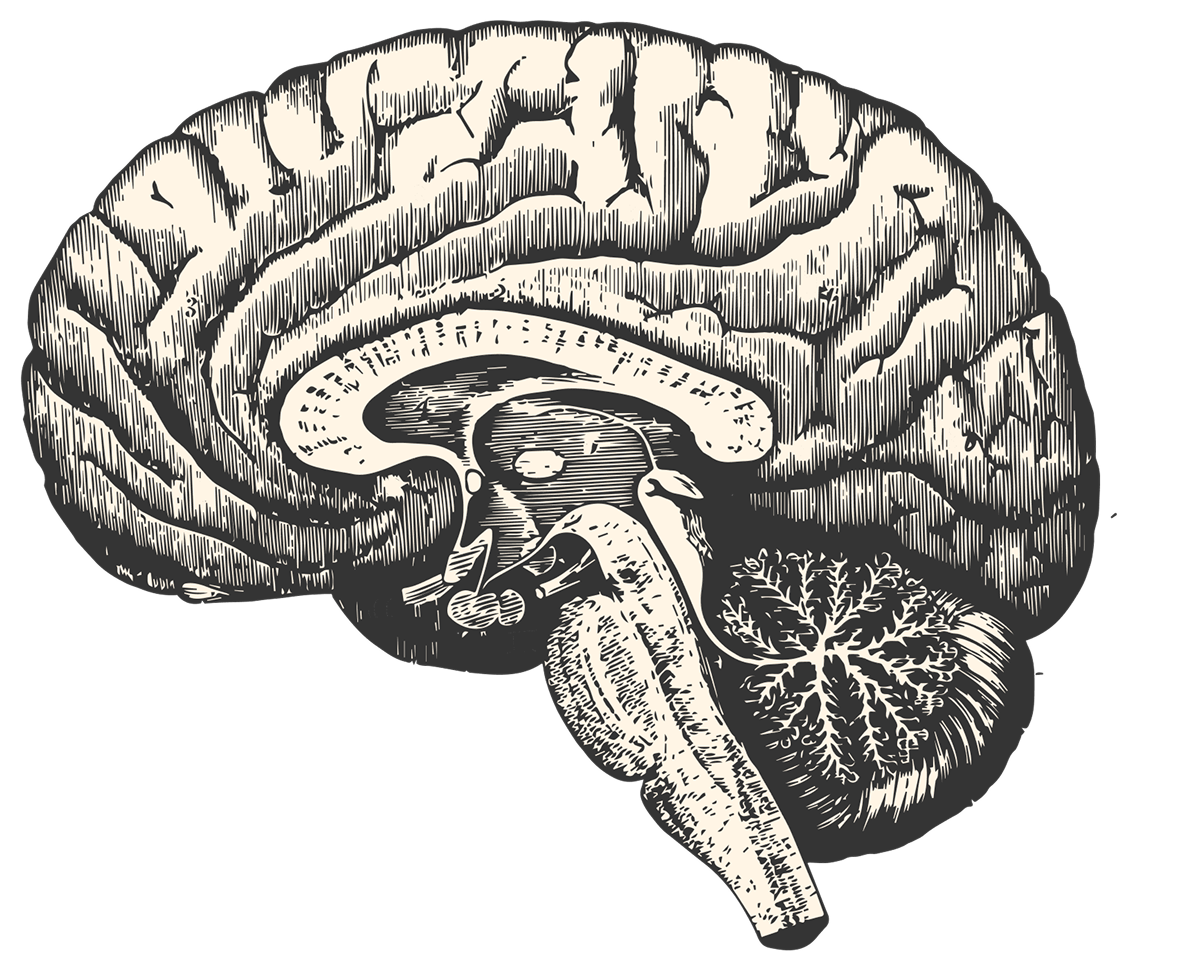
by 1 year
(Net Present Value over decades)
(by 2050)
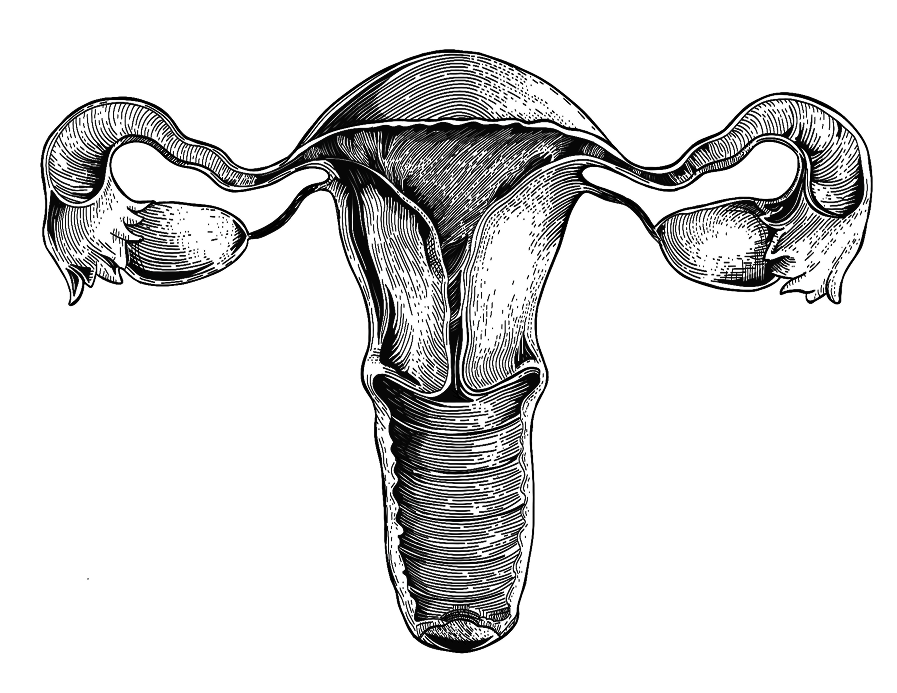
aging by 1 year
(Net Present Value over decades)
(by 2050)
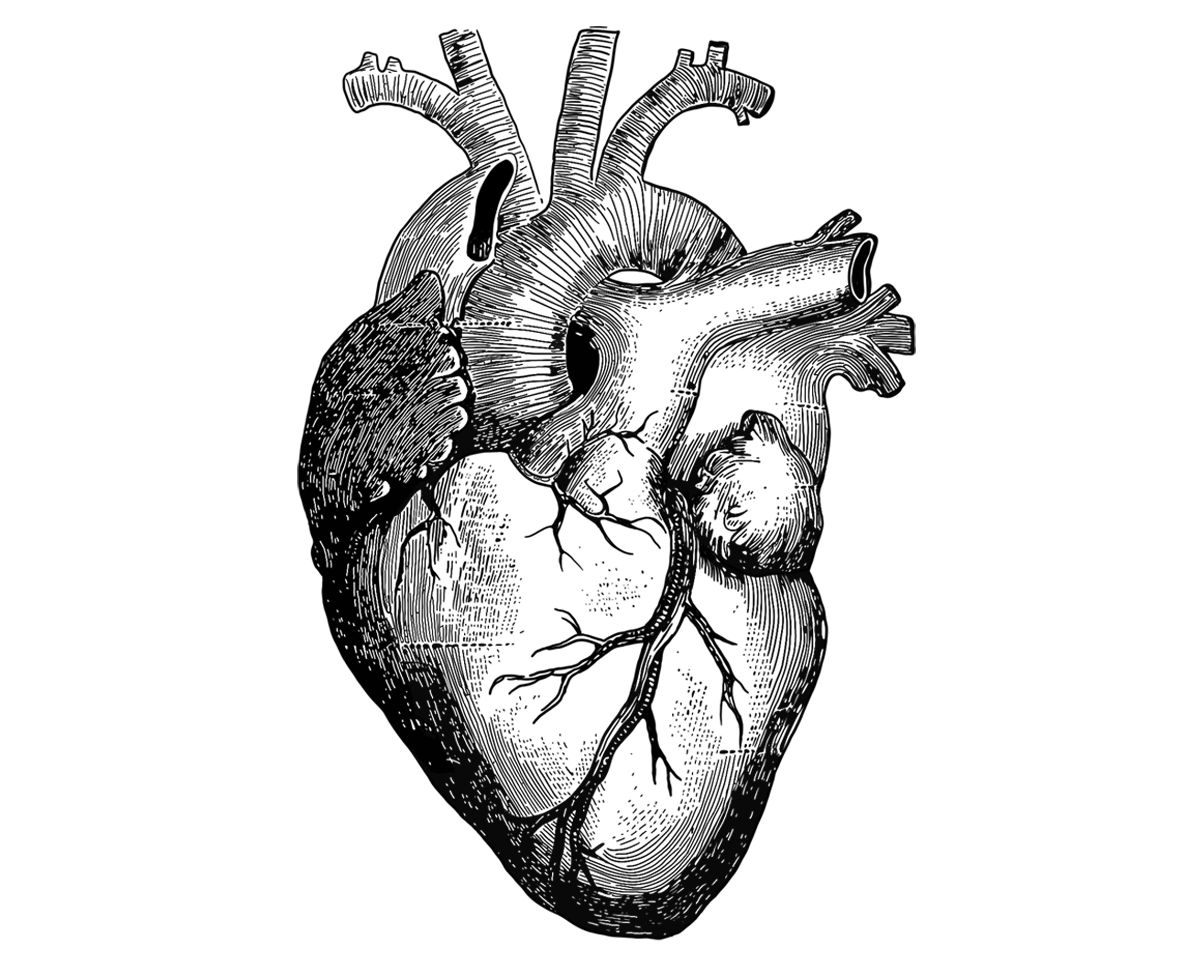
organ supply
(Net Present Value over decades)
(by 2050)
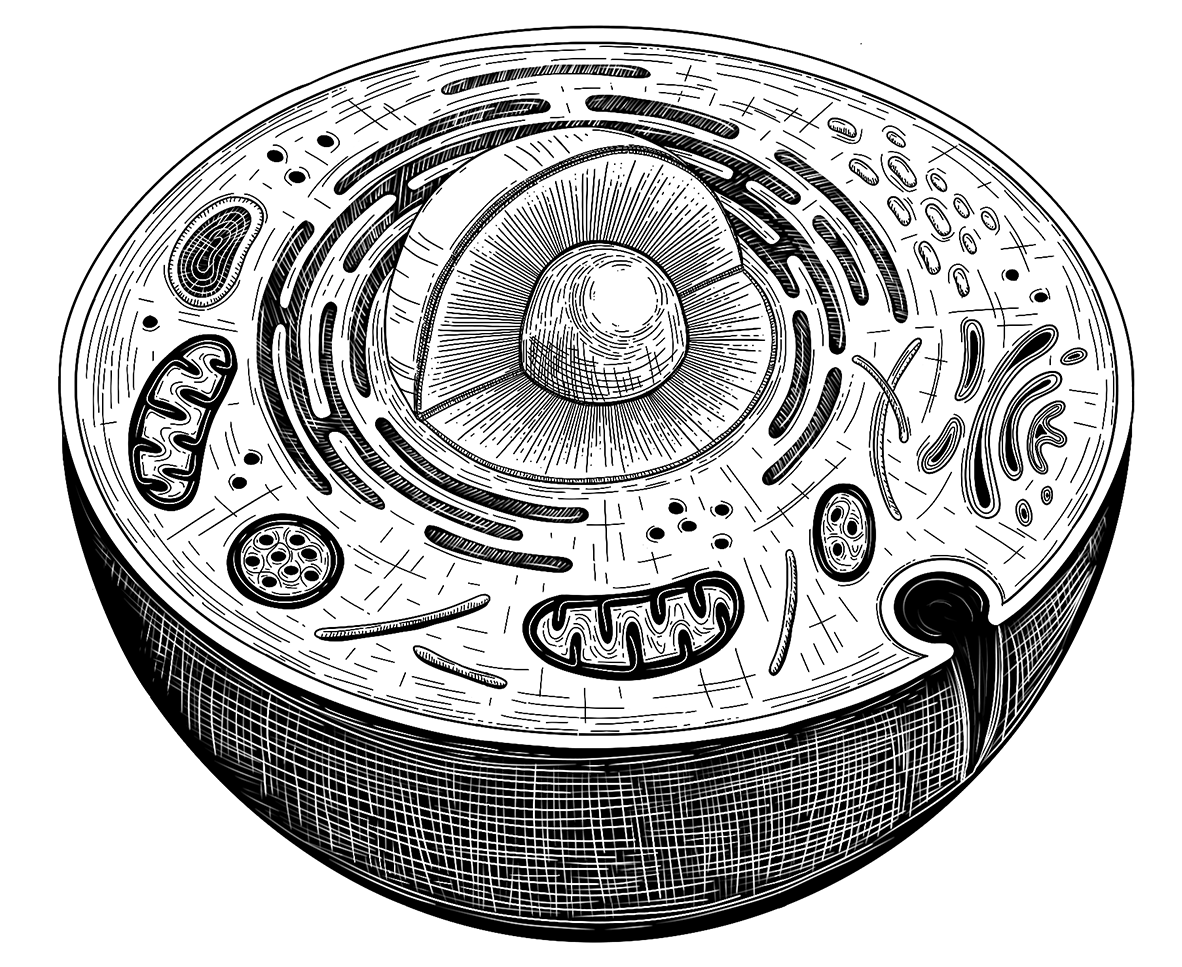
aging by 1 year
(Net Present Value over decades)
(by 2050)
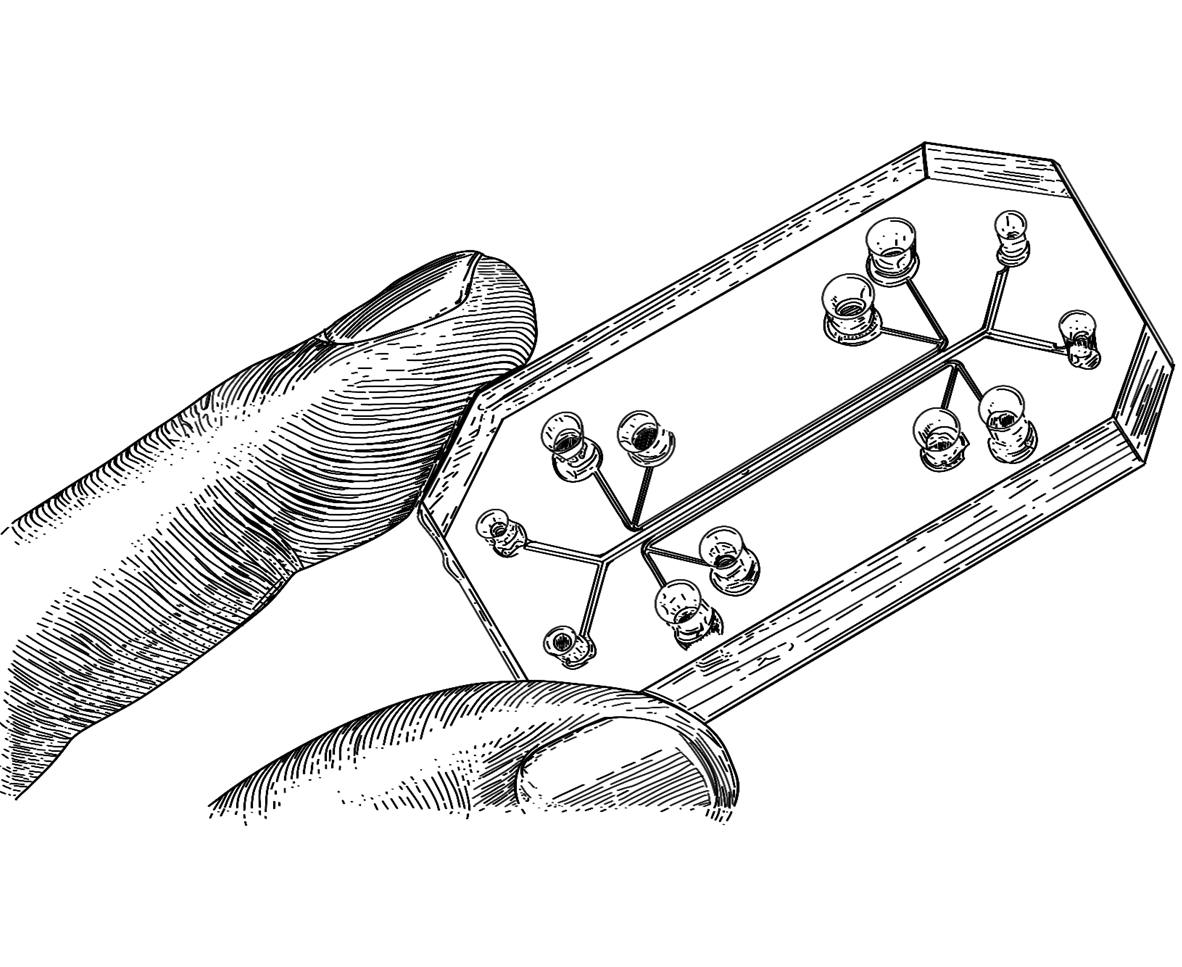
marginally slow aging
(Net Present Value over decades)
(by 2050)
This is the beginning of an open and evolving project. Our goal has not been to predict when a combination of safe, effective, and affordable therapeutics designed to slow aging will be available. Neither has it been to forecast what types of scientific breakthroughs are likely to occur first. Instead, we highlight the value of advancements in science which, if successful, would offer extraordinary returns on investment from a societal standpoint — but for which commercial or institutional incentives have been lacking. Our hope is for you to scrutinize each of our simulation assumptions, and to input your own timelines and views on scientific feasibility, then see returns on investment in terms of lives saved and GDP gains for small R&D advancements in aging biology.
If you feel the parameters in our simulation tool do not allow you to input an important assumption, we would love to hear from you. But we hope you will trust that we have spent much time considering the best way to make our simulations as interactive as possible, while not overwhelming you with too much optionality. We have also spent much time considering how to best translate scientific advancements into economic and demographic outcomes. For instance, we are aware that the mapping of improvements in biological aging through the lens of shifts in mortality, productivity, and fertility rates by age is imperfect. We discuss the limits, rationale, and strengths of our modeling approach in more detail in our technical paper and forthcoming book. Trained economists can also inspect our open-source model (see "About"), though we admit the book may be a necessary companion to fully grasp each model parameter.
If you catch any error in our simulations, please write to us!
Slight improvements in reproductive aging alone would have extraordinary socioeconomic value. Yet we cannot predict that if the U.S. invests, say, $25 billion — half the entire annual budget of the National Institutes of Health as of 2024 — into research on delaying menopause by 10 years, this future would surely exist. Some futures may be millions of dollars and a handful of scientists away, while others may demand billions of dollars and hundreds of tiny technological convergences. This is why we chose not to peg specific investment amounts to each advancement. As Samuel Rodriques, CEO of FutureHouse, shared with us over an email, "the answer to most of these questions is that we simply do not know at this point in time."
We note that the direction of R&D investments is perhaps more important than the funding amount. For instance, heavily funding research on late-stage neurodegenerative diseases (at roughly $3 billion/year as of 2024) has so far produced discouraging results. This is why we advocate for new R&D funding mechanisms by philanthropic foundations, bold federal agencies, and individual donors willing to bet on historically overlooked approaches in science. Some readers may wish to assume a doubling in the existing NIH funding for each R&D area. Others may be interested in forcing this exercise into an apples-to-apples comparison by assuming an equal investment amount to unlock each alternative future. Most scientists we interviewed believe it would take between $1 - $50B to reverse overall biological aging by 5 years - our most ambitious simulation. Only 6% of respondents believe this 5-year simulation would require more than $50B in funding to engineer. Without exception, the simulated returns dwarf any plausible investment amount.
Note: $300B annual ROI = ~1% GROWTH IN U.S. GDP
In a world with competing resources, why invest in aging research?
Say all cancers could be eradicated starting tomorrow. What would this be worth to the U.S. economy? None of our simulations have focused on a scenario as optimistic for aging science. Our most ambitious simulation so far models a 5-year improvement in biological aging — it does not assume we may forever delay all age-related health decline. We have also assumed only 50% of the population would benefit from any given therapeutic. Still, there may not be a ceiling to how many times aging can be therapeutically targeted. This is an important factor when comparing it to returns from alternative R&D areas. But to consider what is possible in the near future, we compare the returns from alternative breakthroughs to a 5-year improvement in biological aging.
Murphy and Topel estimate the value of curing all cancers adds up to about 80$ trillion over several decades (adjusted to 2025 dollars) using Value of Statistical Life.* Using our macroeconomic model and the same discount rate as Murphy and Topel (3.5%), we find slowing aging by just 5 years would add $87 trillion to U.S. GDP.
If all cancers could be wiped off planet Earth tomorrow, this would add between 2 and 3 years to the average life expectancy. Since the median age for a cancer diagnosis is 66, most would-be cancer patients would anyway soon die from other age-related conditions like heart disease, dementia, or a broken rib. Today, only about 1% of all cancer patients are children or adolescents, and the primary risk factor for most cancers remains age. Biological aging is even a neglected factor in select rare diseases (like progeria) and in most severe cases of infectious diseases.The White House recently announced its Cancer Moonshot to “reduce the death rate from cancer by at least 50% over the next 25 years, and improve the experience of people living with cancer.” Research on the biology of aging might just be the most practical way to achieve this goal.
Slight improvements in reproductive aging alone would have extraordinary socioeconomic value. Yet we cannot predict that if the U.S. invests, say, $25 billion — half the entire annual budget of the National Institutes of Health — into research on delaying menopause by 10 years, this future would surely exist. Some futures may be millions of dollars and a handful of scientists away, while others may demand billions of dollars and hundreds of tiny technological convergences. This is why we chose not to peg specific investment amounts to each advancement.
We note that the direction of R&D investments is perhaps more important than the funding amount. For instance, heavily funding research on late-stage neurodegenerative diseases (now at roughly $3 billion per year) has so far produced discouraging results. This is why we advocate for new R&D funding mechanisms like philanthropic foundations, bold federal agencies, and individual donors willing to bet on historically overlooked approaches in science. Some readers may wish to assume a doubling in the existing NIH funding for each R&D area. Others may be interested in forcing this exercise into an apples-to-apples comparison by assuming an equal investment amount to unlock each alternative future. Without exception, the simulated returns dwarf any plausible investment amount.
An Innovation Accelerator to bridge America’s R&D “Valley of Death”
The U.S. remains the global leader in the development of innovative biotechnologies, but faces a persistent bottleneck in bringing many breakthroughs to market. The crucial phase between scientific discovery and private-sector adoption is too often under-resourced. This fragile phase, known as the “innovation Valley of Death,” is where most biotechs fail—and those in aging science are no exception. One solution proposed to us by Dr. Michael Stebbins, former Assistant Director for Biotechnology at the White House Office of Science and Technology Policy, is to establish a new Innovation Accelerator to accelerate progress in aging science: a federally supported, independent fund modeled on In-Q-Tel, the government-backed venture fund that invests in companies producing innovations for the intelligence community. By operating outside of government bureaucracy, this Accelerator can be nimble and invest in early and mid-stage companies developing technologies of national importance—de-risking them for private investment while accelerating their path to commercialization.
Today’s funding gap means that small teams working on difficult problems like aging biology often often have to rely on SBIR/STTR support, which is frequently slow, bureaucratic, risk-averse and sized too modestly to scale transformative ideas. By contrast, the Innovation Accelerator would use modern investment tools—ranging from matching funds to revolving investments backed by returns—and would be empowered to partner with both government and private capital. To protect public interest, companies could be required to keep operations in the U.S. for a fixed period after funding, curbing the offshoring of taxpayer-funded breakthroughs. Dr. Stebbins writes:
“Typically, early and mid-stage innovations are too immature for private capital investors because of the outsized risk inherent to the innovation cycle, have an uncertain regulatory path, or do not fit into an available market. In addition, private capital tends to be more conservative in rough economic times, which dries up the innovation pipeline. While the U.S. government provides significant early-stage discovery funding for innovation through its various agencies, the grant lifecycle is such that after the creation and initial development of new technologies, there are few mechanisms for continued support to drive products to market. It is this period that creates a substantial bottleneck for entrepreneurs where their work is too advanced for the usual government research and development grant funding and not developed enough to draw private investment.”
Those interested in realizing this policy idea can reach out to Dr. Stebbins directly at: mike@mikestebbins.com.
Advance Market Commitments (AMCs) for aging biomarkers
When it comes to preventative health and longevity, private markets have so far mostly optimized for an unproven and unregulated supplements industry. This mismatch between market incentives and social needs suggests public and philanthropic funds and new policy choices are needed to provide, as Nan Ransohoff (founder of climate AMC Frontier) writes, “a stepping stone to a self-sustaining market.” It is possible that well-funded startups in the longevity space will alone validate credible ways to therapeutically target biological aging in the coming years. Yet given the size, importance, and complexity of the problem at hand, a technology-agnostic, company-agnostic mechanism may be necessary to incentivize the creation of the counterfactual futures outlined in this book. The discovery and validation of aging biomarkers and surrogate endpoints particularly suffers from a lack of commercial incentives. As Scannel et al. suggest, any company that discovers and validates predictive biomarkers or surrogate endpoints would receive only a tiny fraction of the socio-economic benefits they unlock. From a purely financial standpoint, the same company would be better off developing drugs for late-stage diseases.
Advance Market Commitments (AMCs) may be a fitting mechanism to close this incentives gap. Operation Warp Speed is sometimes referred to as an AMC, even though the operation was forced to quickly commit to select companies and technologies. The U.S.-guaranteed purchase orders for nearly one billion vaccine doses against Covid-19 ensured that, in a competitive market, even if a small pharmaceutical company (like Moderna) only managed to develop a vaccine slightly after a bigger company (like Pfizer), large profits would remain guaranteed. This has proven effective in steering companies’ direction of their own scarce resources.
Ransohoff explains how “AMCs can send a strong and immediate signal that there is a market for a product, and do so without picking winning technologies at the start.” In the case of research on the biology of aging, this technology- and company-agnostic approach may be critical, since no single group of scientists in 2024 can predict which exact approaches or breakthroughs will be needed to validate, then scale longevity drugs. Good reports have been written on the major bottlenecks in aging research. But as Philip Tetlock has shown with his research on forecasting, specialists do not have a good track record in predicting the exact advancements needed in their own fields. In fact, their judgment is on average poorer than generalists’ in predicting what solutions ultimately succeed.
Previous AMCs have typically promised high budgets for specific problems, without narrowly confining researchers or companies to any single approach to solving it. Used so far for advancements ranging from climate technologies to vaccines for neglected diseases, AMCs can uniquely align market incentives to social needs. AMCs could effectively catalyze a market for real aging drugs, attracting companies large and small to devote vast resources to this historically neglected science. AMCs have ranged from a few hundred million dollars to over a billion dollars. The 2007 AMC for pneumococcal vaccines had a mix of governments and philanthropists pledge $1.5 billion to the cause. Frontier, funded by several for-profit companies and philanthropists to accelerate carbon removal, committed $925 million. Frontier (whose leadership has no vested interests or biases in aging science) even has an open call inviting researchers and funders to brainstorm their AMC idea. AMCs would not be led by one academic laboratory with significant stakes in dictating the rules of grantmaking in their own favor, or with biases towards their own scientific approaches. Instead, they can be structured so that the best solution wins, period.
When it comes to aging biomarkers and surrogate endpoints, a potential AMC may fall within what Ransohof describes as a “Scenario-2 AMC,” where the problem “is of a scale where a single AMC can only kick-start it.” We encourage interested readers to reach out to Frontier to fund or catalyze an AMC for therapeutics that slow biological aging, whether by directly enabling existing trials like TAME or incentivizing the discovery and validation of predictive aging biomarkers.
To our knowledge, ours is the only attempt so far at measuring the effects of targeting biological aging on Gross Domestic Product (GDP). We decided to fill this gap in the literature and to focus on GDP for two major reasons. First, GDP is directly impacted by the number of working-age adults. Second, GDP often correlates with important measures of well-being. GDP growth does not directly indicate whether a population’s quality of life is improving, but it offers important insights. This is largely because in this first quarter of the 21st century, work is a useful proxy for health. (Perhaps as good as LDL cholesterol for heart health — which is to say, imperfect, but helpful.)
Of course, GDP is an incomplete measuring stick. At times, GDP may increase as a result of overall lower well-being. At other times, changes in GDP undermine the effects of experiences like grief. For instance, a focus on GDP may overlook how working-age adults or unpaid caretakers might happily trade decreases in GDP for, say, a cure for their loved one’s Alzheimer’s. There is much that GDP as a metric cannot measure, and much that it can.
In our forthcoming book, we survey the long and rich history of papers on the economics of longevity, and discuss the pros and cons of different methodologies that measure non-market outcomes, including Value of Statistical Life (VSL), Willingness to Pay (WTP), and Quality Adjusted Life Years (QALYs). We also take a closer look at the effects of each R&D area on GDP per capita. (All our simulations — even the one on reproductive aging — result in positive GDP per capita returns).
For readers interested in decoupling the value of human life from human productivity, we recommend the 2021 Nature paper by Scott et al. “The economic value of targeting aging.” There, the authors use VSL to more abstractly measure the value of human life — whether or not the humans in question are working. For instance, newborns don’t add to GDP in the short term. Our model takes into account their formal contributions to GDP once they enter the workforce, and once they have newborns who decades later increase labor supply, too. But using VSL, newborn life years can be measured as immediately having economic value. Both methodologies are helpful. Our approach has been to measure more direct impacts on the U.S. economy, in the short and long run.
(The two graphs below are based on World Bank data.)
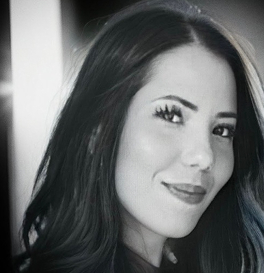
Raiany Romanni is a researcher and writer with a decade-long focus on human longevity. Before leading this project, she helped design and launch the $101M Healthspan XPRIZE, where it became clear to her that economic and demographic datasets were needed to justify public and private investments in longevity. She oversaw the writing, team building, fundraising, design, and overall execution of this project while based at The Amaranth Foundation.
Raiany is broadly interested in understanding why secular humans like to narrate death and aging as good things — as if they’d been designed for the good of our species. She's also interested in quantifying the effects of this narrative on economies and people. Her upcoming book with Harvard University Press is titled Silver Linings: The Ethics and Economics of Redesigning Aging. Raiany is now based at the Wyss Institute for Biologically Inspired Engineering at Harvard University.
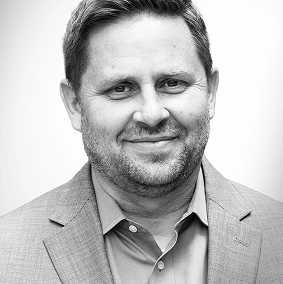
Richard Evans is a Senior Economist at the Abundance Institute, and an Advisor to QuantEcon. He is also a Non-Resident Fellow at the Brookings Institution. Before that, he founded the Open Source Economics Laboratory, and was a Fellow at the Becker Friedman Center at the University of Chicago. He has provided macroeconomic modeling for the United Nations, the European Commission, and the World Bank.
Richard is a co-developer of the open-source economic model used in this project, and a co-author in the book that resulted from this work. Richard and Jason (right) were the two lead economists in this project.
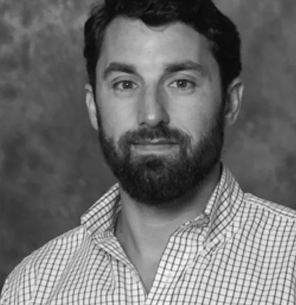
Jason deBacker is Associate Professor of Economics at the Darla Moore School of Business, and President of the Policy Simulation Library Foundation. He is also a consultant for the World Bank and the United Nations, and a Non-Resident Fellow at the Brookings Institution.
Jason is a co-developer of the open-source economic model used in this project, and a co-author in the book that resulted from this work. Jason and Richard (left) were the two lead economists in this project.
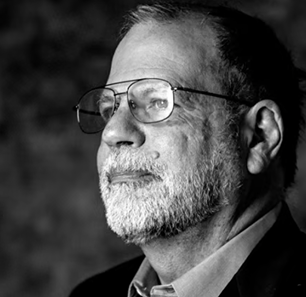
Tyler Cowen is Holbert L. Harris Professor of Economics at George Mason University and Director of the Mercatus Center. He was named in an Economist poll as one of the most influential economists of the last decade. Bloomberg BusinessWeek dubbed him "America's Hottest Economist." Foreign Policy magazine named him one of its "Top 100 Global Thinkers."
Tyler was the first economist to accept working with Raiany on this project, advising her on its direction and writing.
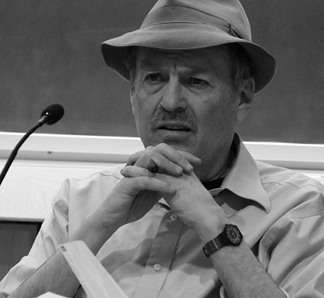
Richard Freeman holds the Herbert Ascherman Chair in Economics at Harvard University. He is a Research Associate at the National Bureau for Economic Research, and Co-Director of the Harvard Law School Center for Labor and a Just Economy.
Richard made many valuable contributions to the simulations and arguments in this project.
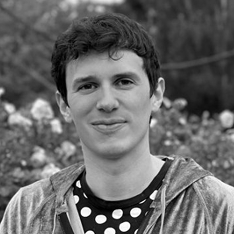
James Fickel is an investor and futurist. He is the founding donor of The Amaranth Foundation and the The Enigma Project at Stanford School of Medicine. He has backed several labs at Harvard, MIT, and Stanford.
James was this project’s primary funder. This research would not exist without James and the team he assembled at The Amaranth Foundation.
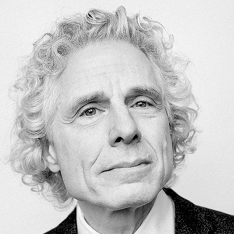
Steven Pinker is the Johsntone Professor of Psychology at Harvard University. His bestselling books include Enlightenment Now and The Blank Slate. He has been named Time magazine’s “100 Most Influential People in the World Today.”
Steve advised an earlier version of this project, Raiany Romanni’s PhD dissertation on the Ethics and Economics of Human Longevity.
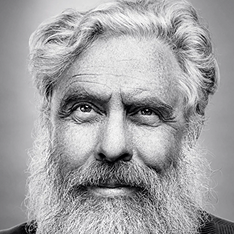
George Church is the Robert Winthrop Professor of Genetics at Harvard Medical School. He is Director of the National Institutes of Health Center of Excellence in Genomic Science, and has received numerous awards including election to the National Academy of Sciences and Engineering. He has been named Time magazine’s “100 Most Influential People in the World Today.”
George advised an earlier version of this project, Raiany Romanni’s PhD dissertation on the Ethics and Economics of Human Longevity.
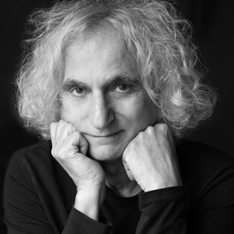
Oded Galor is the founder of Unified Growth Theory, best-selling author of The Journey of Humanity: The Origins and Wealth and Inequality, and the Herbert Goldberger Professor of Economics at Brown University.
Oded advised an earlier version of this project, Raiany Romanni’s PhD dissertation on the Ethics and Economics of Human Longevity.
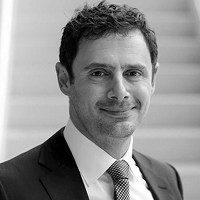
Eric Budish is the Paul G. McDermott Professor of Economics and Entrepreneurship at the University of Chicago, Booth School of Business.
Eric generously offered several rounds of feedback on this project, and helped workshop different ideas and methodologies used in it.
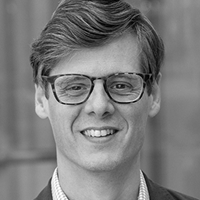
Eli Dourado is the Head of Strategic Investments at the Astera Institute. Before that, he was the Chief Economist at the Abundance Institute, and a Senior Research Fellow at the Center for Growth and Opportunity. He focuses on the hard technology and innovation needed to drive large increases in economic growth.
Eli offered generous feedback and advice on the earliest iterations of this project, and helped recruit the team to execute on it.
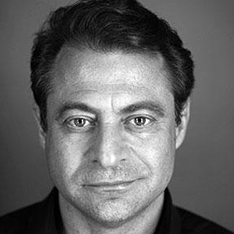
Peter Diamandis is the Chairman of the XPRIZE Foundation. During the launch of the $101 Healthspan XPRIZE, it became clear to Peter as well as other prize funders and designers that more research on the economics of longevity was needed.
Peter offered invaluable encouragement and support for this project.
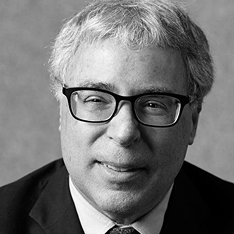
Nir Barzilai is the Director of the Institute for Aging Research at the Albert Einstein College of Medicine, President of The Academy for Health & Lifespan Researchand, a Board Member at the American Federation for Aging Research. Nir also led the design of the TAME trial, whose socio-economic value is simulated in Future 4. ("We can measure & marginally slow aging.)
Nir offered support and encouragement for this project.
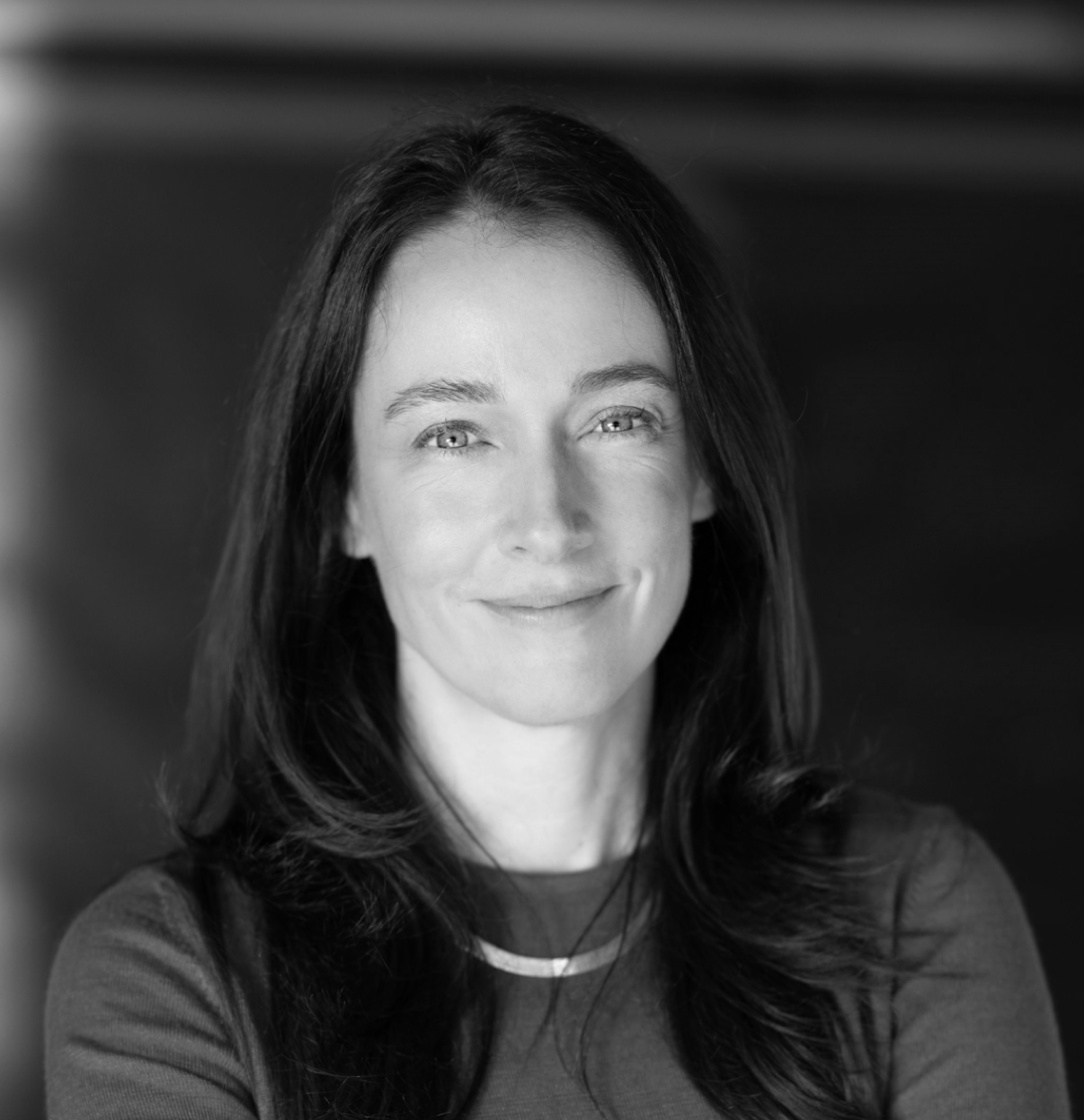
Kristen Fortney is the CEO of BioAge, a clinical-stage biotechnology company developing a pipeline of therapies to extend healthy lifespan by targeting the molecular causes of aging. She is also an advisor for The Amaranth Foundation.
Kristen offered feedback on many sections in this project.
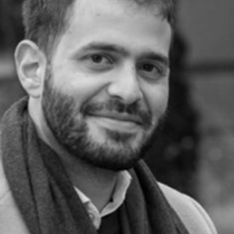
Santi Ruiz is the senior editor at The Institute for Progress and author of the Statecraft newsletter.
Santi helped edit an earlier version of this project.
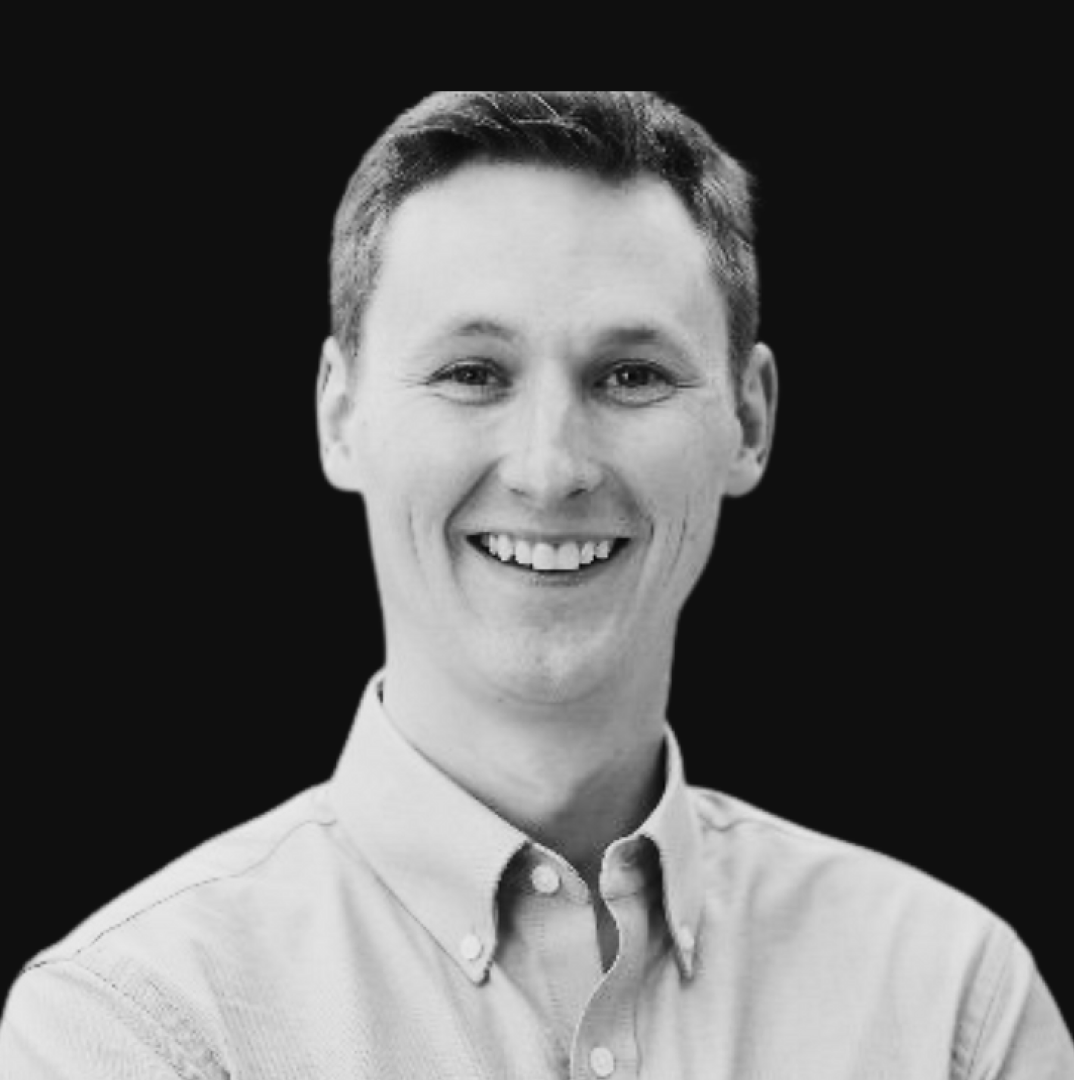
Alex Colville is an Advisor for the Amaranth Foundation and General Partner at age1, a venture capital firm that invests in early-stage biotechs focused on longevity. Before that, he completed his PhD and post-doctoral work in Genetics at Stanford School of Medicine.
Alex offered valuable feedback on early iterations of this project, including the idea of mapping what might be the first-ever human clinical trial with an aging endpoint, like the TAME trial.
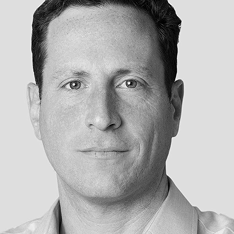
Brian Bergstein is the Ideas Editor at The Boston Globe. He was previously the Editor at Large at the MIT Technology Review, and the founding editor of NEO.LIFE, a biotechnology-focused publication. Before that, he was a Knight Science Journalism Fellow at the Massachusetts Institute of Technology.
Brian helped brainstorm this project’s publication strategy and content.
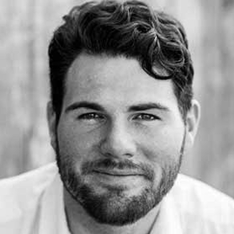
Dane Gobel is a Co-founder and Program Director of the Methuselah Foundation.
Dane and The Methuselah Foundation offered invaluable feedback, funding, and support for this project.
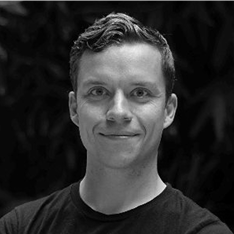
Martin Borch Jensen is the CSO at Gordian Biotechnology and President at Norn Group. Before that, he was an academic working on a range of cellular mechanisms involved in aging: mitochondrial function, NAD metabolism, DNA damage signaling and other stress responses.
Martin offered substantial feedback and comments on several parts of this project.
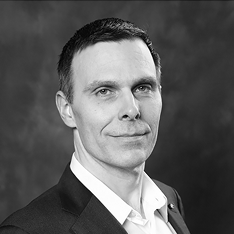
Matt Kaeberlein is the CEO of Optispan and Co-Director of the Dog Aging Project. He served as founding Director of the University of Washington Healthy Aging and Longevity Research Institute, Director of the NIH Nathan Shock Center of Excellence in the Basic Biology of Aging, CEO and Chair of the American Aging Association, and received his PhD in Biology from MIT.
Matt offered extensive comments on many sections in this project.
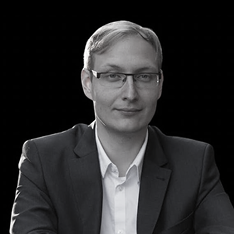
Samo Burja is a Senior Research Fellow in Political Science at the Foresight Institute and President of Bismarck Analysis. He also chairs the editorial board of Palladium Magazine.
Samo and the Bismarck team helped brainstorm the first iterations of this project. Samo also helped edit early versions of the report.
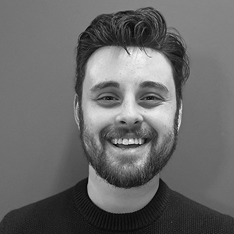
Rohan Krajeski is a PhD Candidate in Biotechnology (Neuroscience) at the University of Cambridge. Before that, he was a researcher at the MIT Department of Brain and Cognitive Sciences, and a consultant for the Amaranth Foundation.
Rohan offered extensive comments on many sections in this project.
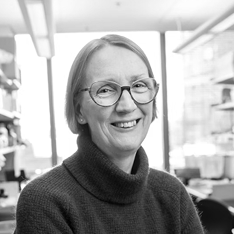
Jeantine Lunshof is Head of Collaborative Ethics at the Wyss Insitute for Biologically Inspired Engineering at Harvard University. She mentored Raiany Romanni’s Master’s in Bioethics capstone project at Harvard Medical School, titled “A New Ethics for Aging.”
Jeantine offered feedback on select portions of this project with moral and philosophical underpinnings.
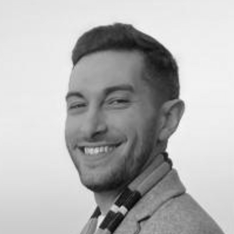
Joshua Daniel Crapser is a post-doctoral fellow in neurology and neurological sciences at Stanford School of Medicine. He was previously a consultant for the Amaranth Foundation.
Joshua offered significant feedback on the brain and organ aging sections in this project, and helped estimate current NIH investment amounts in brain aging.
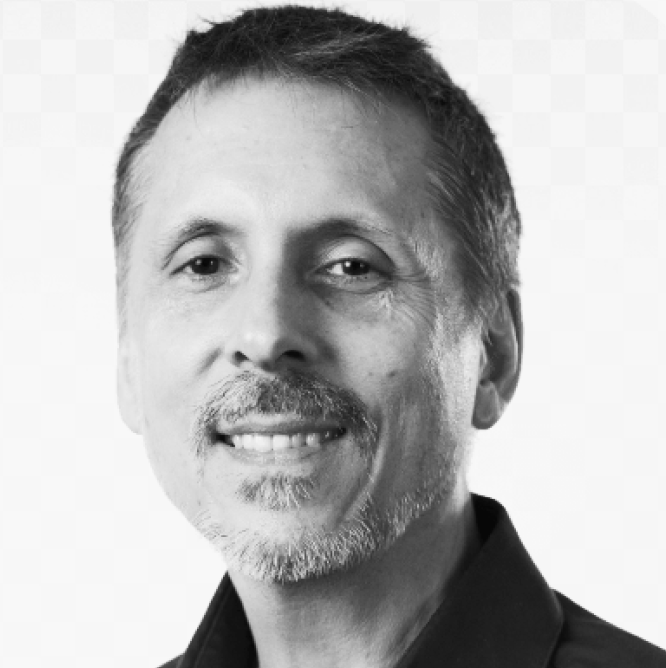
Jean Hebert is a Program Manager in Health Sciences Futures at ARPA-H. Before that, he was a Professor in Neuroscience and Genetics at Albert Einstein School of Medicine.
Jean inspired and offered extensive feedback on this project’s section on increased organ supply, which is a nod to his book Replacing aging.
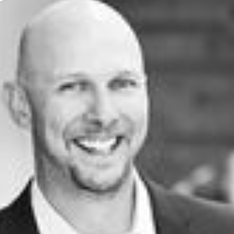
Michael Stebbins is a geneticist and public policy expert. He served in the Obama Office of Science and Technology Policy and was the architect and driving force behind the creation of ARPA-H.
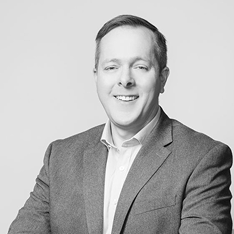
Stuart Buck is the Executive Director of the Good Science Project. Before that, he was Vice President at Arnold Ventures. He helped launch the Center for Open Science, Vivli, the Stanford Center for Reproducible Neuroscience, the Yale Collaboration on Research Integrity and Transparency, and the Evidence-Based Medicine DataLab at Oxford. Stuart received his J.D. from Harvard Law School.
Stuart offered generous feedback and encouragement on this project.
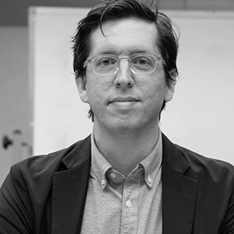
Nathaniel Hendrix is a Researcher and Data Analyst at the American Board of Family Medicine. He is also a Research Affiliate at Stanford University, School of Medicine. He was previously a post-doctoral fellow in health economics at Harvard University, T.H. Chan School of Public Health.
Nathaniel helped oversee the data analysis for many simulations in this project. He also helped synthesize our lists of R&D advancements that remain underserved by commercial incentives.
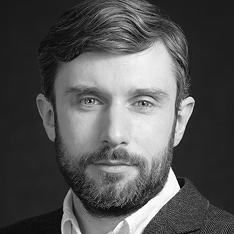
Andrew Steele is a scientist, writer and campaigner based in Berlin, and author of Ageless: The new science of getting older without getting old. He holds a PhD in Physics from the University of Oxford, after which he completed several post-doctoral fellowships on aging biology.
Andrew offered feedback on many graphs and arguments in this project.
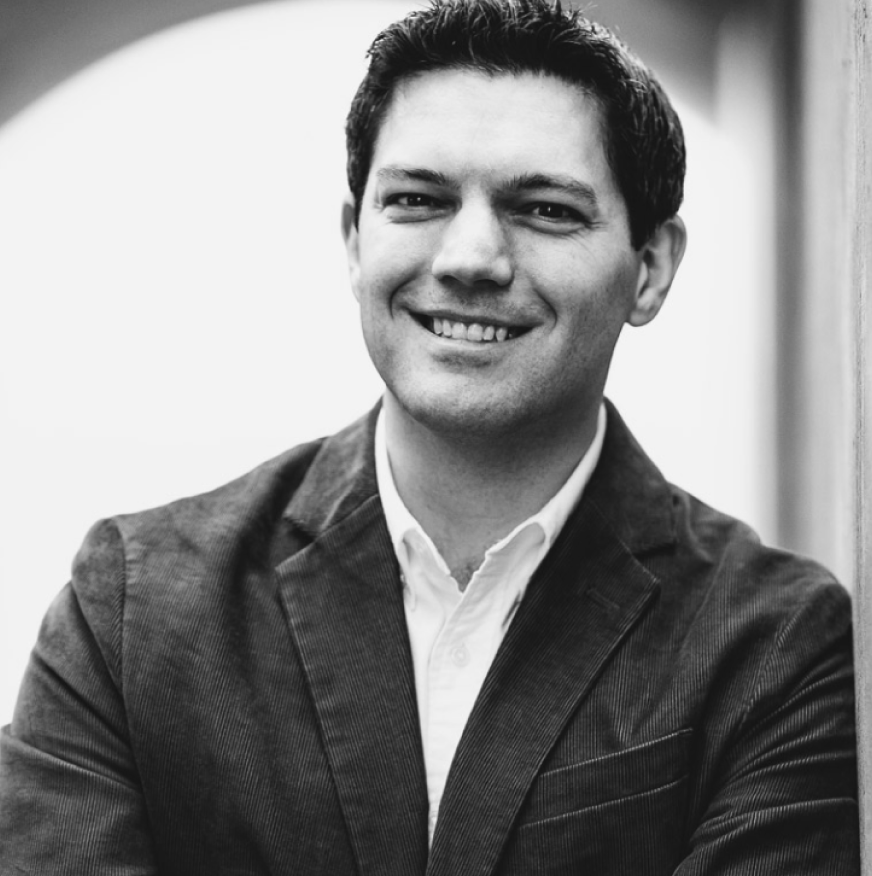
Jason Crawford is founder of the Roots of Progress Institute, and a consultant to Our World in Data.
This project was workshopped at the Roots of Progress Institute, where Raiany was a Fellow, and where it benefited from feedback from several Roots of Progress fellows and advisors, including Jason.
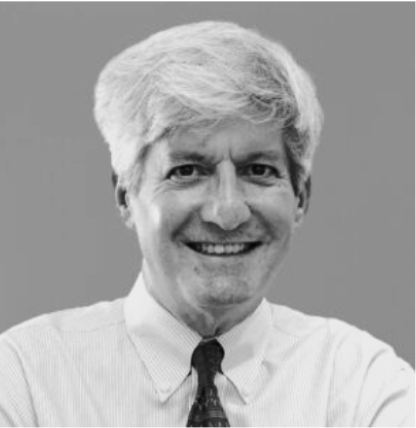
Tom Kalil is the CEO of Renaissance Philanthropy. Tom served in the White House for two presidents (Obama and Clinton) and in collaboration with his team worked with the Senate to give every federal agency the authority to support incentive prizes for up to $50 million. Tom also designed and launched dozens of White House science and technology initiatives, including the $40 billion National Nanotechnology Initiative, announced by President Clinton and The BRAIN Initiative, announced by President Obama.
Tom offered encouragement on the idea to quantify the value of more reliably measuring biological aging.
To arrive at the 5 R&D areas and tables of 50 possible scientific advancements outlined in this project, we interviewed 102 scientists and held workshops with many of them. For instance, our section on brain aging was inspired by an Amaranth-sponsored workshop held in San Francisco with several leading neuroscientists. (See the white paper that resulted from this workshop, led by neuroscientist Rohan Krajeski.) Our prerequisite for selection of each research area has been low commercial and institutional incentives, but potential for high socioeconomic returns.
Most of the scientists below completed a typeform survey with 7 questions ranging from timelines for longevity drugs to funding required for specific advancements. A few scientists (including George Church, Samuel Rodriques, and Blake Byers) were instead interviewed in person, over email, or over a video call. Our Harvard University Press book will cover these interviews and conversations in more detail. Dozens of names in this list of scientists interviewed also offered feedback on earlier versions of this project.
Select data findings from our interviews with 102 longevity scientists:
72% of respondents believe that slowing aging by 5 years is already possible today with low-tech solutions like exercise and/or existing therapeutics.
77% of respondents believe we will be able to slow biological aging by 30 years within 20 - 40 years.
86% of respondents believe there is no ceiling to how much we can extend healthy lifespan.
51% of respondents believe it would take an investment ranging between $1 - $50 billion to arrive at a future where biological aging can be reversed by 5 years. 43% of respondents believe this could be done with roughly $1 billion in funding, since funding direction is more important than funding amount. Only 6% of scientists believe it would take more than $50B for the most ambitious of our simulations: a 5-year reversal in biological aging.
53% of respondents believe human aging will be managed mostly via intracellular/epigenetic therapeutics. 31% believe a combination of therapeutics and “replacement” approaches (e.g. replacing the extracellular matrix to improve brain aging) will be needed.
78% of respondents have no moral concerns about longevity research, since “saving lives is a moral imperative.” 21% of scientists responded that “some spill-over effects of longevity (e.g. environmental impacts from larger populations) may prove difficult to solve, but they will be worth solving.”
In the final open question, respondents shared one R&D area in aging biology they believe is currently underserved by market incentives, but could yield extraordinary socio-economic returns if better supported by public/philanthropic funds. The result is a list of R&D approaches below each simulated scenario. (We supplemented these responses with our own research to make up roughly 10 sample R&D projects for each future.)
By Raiany Romanni
Jason DeBacker & Richard W. Evans
(Book under contract with Harvard University Press)
Working paper on the economics of longevity
By Jason DeBacker, Richard W. Evans, and Raiany Romanni
Federation of American Scientists policy memo By Raiany Romanni, Enlli Lewis, and Josh Morrison
These futures won’t build themselves. If you want to enable one or several of these simulated futures — where thousands or millions of lives can be saved or improved — we want to help.
POLICYMAKERS
See these two FAS policy memos, play with our interactive simulation tool, read this paper, or reach out to Raiany Romanni with any questions and she’ll respond within 24 hours.
Researchers
Economists: See each parameter in our open-source model here; in our technical paper, and in our upcoming book. Write to us if you’d like to collaborate on spin-off projects, or to critique/build upon our modeling assumptions. Scientists: See our pre-print or our forthcoming book for a more detailed discussion of each R&D area, including a detailed mapping of the clinical trials (e.g. TAME) discussed.
Philanthropists/investors
[In progress]